Welfare in Farmed Decapod Crustaceans, With Particular Reference to Penaeus vannamei
- 1Institute of Aquaculture, University of Stirling, Stirling, United Kingdom
- 2Ryan Institute, School of Natural Sciences, National University of Ireland Galway, Galway, Ireland
- 3Institute of Biodiversity Animal Health and Comparative Medicine, University of Glasgow, Glasgow, United Kingdom
The farming of decapod crustaceans is a key economic driver in many countries, with production reaching around 9.4 million tonnes (USD 69.3 billion) in 2018. These efforts are currently dominated by the farming of Pacific whiteleg shrimp, Penaeus vannamei, which translates into approximately 167 billion farmed P. vannamei being harvested annually. Further production growth is expected in the future and hence the need for more research into its health and welfare is required. Herein, from an extensive survey of the available literature, we scrutinise farming practices and the challenges associated with the production of P. vannamei from an animal-centric welfare perspective (1), we propose potential welfare indicators (2) and we critically review current scientific evidence of sentience in penaeid shrimp among other commercially important decapods (3), since it is plausible that in the near future not only the largest, but in fact all decapod crustaceans will receive welfare protection. This review highlights that despite the wide knowledge on crustacean stress physiology and immunology as well as disease control, still little is known about some key parameters related to the five welfare dimensions. We recommend that further research should focus on developing a systematic integrated welfare assessment encompassing all the different aspects of the crustaceans farming and life cycle up to slaughter. Furthermore, direct and indirect species-specific operational welfare indicators should be developed for all decapod crustaceans currently farmed, similar to the ones suggested in this review for P. vannamei.
1 Introduction
True prawns belonging to the family Penaeidae comprise more than 400 described species and include commercially extremely valuable species such as the giant tiger prawn (Penaeus monodon) and the Pacific whiteleg shrimp (P. vannamei, synonym Lipopenaeus vannamei). As a typical example of their biology, P. vannamei is native to the Eastern Pacific coast from Sonora, Mexico in the North, through Central and South America as far South as Tumbes in Peru, in areas where water temperatures are normally >20°C throughout the year. They occupy tropical marine habitats, where adults mature at 6-7 months and live and spawn in the open ocean. The larval stages are carried towards the shore by tidal currents, and postlarvae move further inshore to spend their remaining pre-adult stages in coastal estuaries, lagoons or mangrove areas (FAO, 2009).
The artificial rearing of a penaeid species for aquaculture can be traced back to more than 70 years ago with the rearing of Kuruma prawn P. japonicus larvae (Hudinaga, 1935; Hudinaga, 1942). However, while initial work was performed in this species, rearing and developmental work especially during the 1960’s and 1970’s expanded quickly in other geographical areas for various penaeid species (Cummings, 1961; Elwald, 1965; Muthu et al., 1974; Thomas et al., 1974). From a commercial production perspective, the work by Hudinaga (Hudinaga, 1966; Hudinaga, 1967) is considered the basis from which the penaeid shrimp industry developed.
The first captive spawning of P. vannamei was achieved in Florida in 1973 from nauplii shipped from a wild-caught mated female from Panama. Following the development of intensive breeding and rearing techniques, the discovery that unilateral eyestalk ablation and adequate nutrition promote maturation, commercial culture of P. vannamei began from the mid-1970s in South and Central America and subsequently in mainland United States of America, with strict quarantine laws or bans to prevent importation of exotic pathogens with new stocks. Although a non-indigenous species in Asia, from the beginning of the millennium P. vannamei has become the dominant species in penaeid shrimp aquaculture in many Asian countries, notably China, Vietnam, Thailand and India, replacing local species such as P. monodon.
In 2018, the total aquaculture production of crustaceans was estimated to be 9.4 million tonnes (USD 69.3 billion) (FAO, 2020). Of this production, more than 50% is accounted for by the farming of P. vannamei (4.96 million tonnes) while P. monodon production is around 8% (750K tonnes). The main producing countries are now based in the Asia-Pacific region (80% production) led by China, while in Latin America the main producing country is Ecuador with 600K tonnes farmed in 2019. While the production is dominated by these two species, knowledge on rearing methods is available for many penaeid species including P. aztacus, P. chinensis, P. merguiensis, P. duorarum, P. japonicus, P. orientalis, P. setiferus, P. stylirostirs and P. indicus among others (see for example Cook and Murphy, 1969; Heng and Rui-yu, 1994). At the time of writing, the only complete genomes available for the family Penaidae are P. vannamei and P. monodon (Zhang et al., 2019; Uengwetwanit et al., 2021). However, given the importance of biodiversification from a food security context (Metian et al., 2020) it is possible that this situation will change in the future and necessary tools and SPF-free seed will be available for other species.
While growth in this sector has been very significant, the sector has suffered from a number of production challenges, mostly driven by disease (see Evaluation of Key Challenges Associated With the Production P. vannamei From a Welfare Perspective). In fact, the main driver for the introduction of P. vannamei to Asia was the perceived differences in its disease susceptibility to white spot syndrome virus (WSSV) compared to P. monodon (Funge-Smith and Briggs, 2003). However, incidences of disease in P. vannamei have flourished with the development of the industry and major crises have been experienced all over the world (Linda et al., 2005; Kumar et al., 2014). In this context, diseases such as acute hepatopancreatic necrosis disease (AHPND), hepatopancreatic microsporidiosis (HPM), hepatopancreatic haplosporidiosis (HPH), aggregated transformed microvilli (ATM) and covert mortality disease (CMD) have been added to the list of previous known threats to the commercial production of P. vannamei, such as white spot disease (WSD), yellow head disease (YHD) and infectious myonecrosis (IMN) (see review Thitamadee et al., 2016). Disease of course plays a significant role in animal welfare. In fact, the word ‘dis-ease’ refers to a state that lacks ‘ease’ or well-being. Thus, one of the outcomes of poor welfare is expressed in the form of disease (Butterworth and Weeks, 2009).
1.1 The ‘Five Domain Model’ as a Framework for Animal Welfare Assessment
While it is clear the importance that disease place within the context of animal welfare, health is only one of the animal welfare pillars within the so-called ‘Five Domains Model’ (Mellor and Reid, 1994). In this model, good welfare or well-being is described as the state that is manifest in an animal when its (1) nutritional, (2) environmental (physical environment), (3) health, (4) behavioural and (5) mental needs are met. The first four domains focus on the animal’s physiological and behavioural needs and encompass pathophysiological disturbances caused by nutritional, environmental and health-related issues. According to Mellor and Beausoleil (2015) once these factors are assessed their anticipated consequences are assigned to the (5) ‘mental’ domain and it is these affective experiences that determine the animal’s welfare state (Mellor et al., 2009). These five domains are essentially equal to the ‘five freedoms’ formulated by the Farm Animal Welfare Council in a number of countries, including the UK (Mellor and Reid, 1994).
From a more fundamental perspective, the term welfare is intrinsically linked with the absence of suffering, which include anxiety, fear, pain and distress and more recently increasingly linked to the promotion of positive states (Mellor and Beausoleil, 2015). However, these experiences, according to most national and international policy frameworks, only apply to animals that are sentient as non-sentient animals are not capable of feeling.
Therefore, in this review we will evaluate the extent to which the five domains of welfare are being met by current commercial practices in the key penaeid commercial species, P. vannamei, and propose future developments that are needed to identify relevant indicators for the evaluation of the welfare of this species when cultured. We will also assess the current evidence for sentience in penaeid and, by extension, other decapod crustacean species, when relevant. We believe these are important considerations per se and especially if increased legislative protection on these animals is to be implemented.
2 Evaluation of Key Challenges Associated With the Production of P. Vannamei from a Welfare Perspective
2.1 Maturation of Female Broodstock and Current Production Practices: Eyestalk Ablation
In natural environments, P. vannamei spawn under specific environmental cues (a combination of optimal water temperature, salinity and dissolved oxygen mainly) stimulating ovary development and spawning by neurosecretory centres (Calderon-Perez et al., 2007). In production systems, parameters such as water temperature, salinity, and dissolved oxygen, nitrogen compounds, photoperiod, light intensity, maturation room and tank characteristics, and diet may be manipulated and adjusted (Browdy, 1998; Whetstone et al., 2002; Jorry, 2012). In general, P. vannamei should be held for maturation under specific conditions that include low light (10-30% of natural or artificial light), ideally with a system to control photoperiod (maintained at about 10-12 dark and 12-14 hours light) (Treece and Fox, 1993; FAO, 2003). Noise (particularly loud or intermittent noise), movement and other disturbances should be kept to a minimum level. Maturation tanks should be round (but in Asia square or rectangular tanks are also used), dark-coloured, smooth sided, and of approximately 3-5 m diameter (FAO, 2003). Stocking densities of around 6–15 shrimp m-2, with a male to female ratio of 1:1, 1:1.5 or 1:2 are optimal. Water temperature, salinity, dissolved oxygen, pH, alkalinity and ammonia are usually maintained at the range of 28 – 29 °C, 30 – 35 ppt, ≥4 mg L-1, 8 – 8.2, ≥100 mg L-1 CaCO3 and < 1 mg L-1 respectively (FAO, 2003). It is also important to make sure that the broodstock have good nutritional status and appropriate size (at least 30 g and >40 g for male and female, respectively), age and origin (i.e., grow-out conditions and genetic background) (Racotta et al., 2003).
The external factors mentioned above are considered to improve success in inducing sufficient egg production to meet commercial schedules, but insufficient to ensure consistent and predictable production scheduling, leading the P. vannamei sector to continue reliance on manipulating the endocrine system of penaeids using eyestalk ablation to improve reproductive performance.
Ablation of eyestalks is broadly used in commercial hatcheries as a crude method of hormonal manipulation to induce maturation and spawning in P. vannamei. It involves the removal or constriction (through cutting, cauterizing or tying) of one (unilateral) or two (bilateral) eyestalks to reduce the level of gonad inhibiting hormone (GIH/MO-IH) which is produced by the X-organ and sinus gland complex situated in the optic ganglia of the eyestalk (Kang et al., 2014; Wang et al., 2019; Magaña-Gallegos et al., 2021). Eyestalk ablation is important as ovarian maturation of P. vannamei, is controlled by GIH hormone and is presumed to inhibit vitellogenesis (Kang et al., 2014). Thus, the eyestalk ablation accelerates ovarian maturation in the female, resulting in spawning (Palacios et al., 1999a). However, from a welfare perspective, both unilateral and bilateral ablations have detrimental effects on females (Table 1).
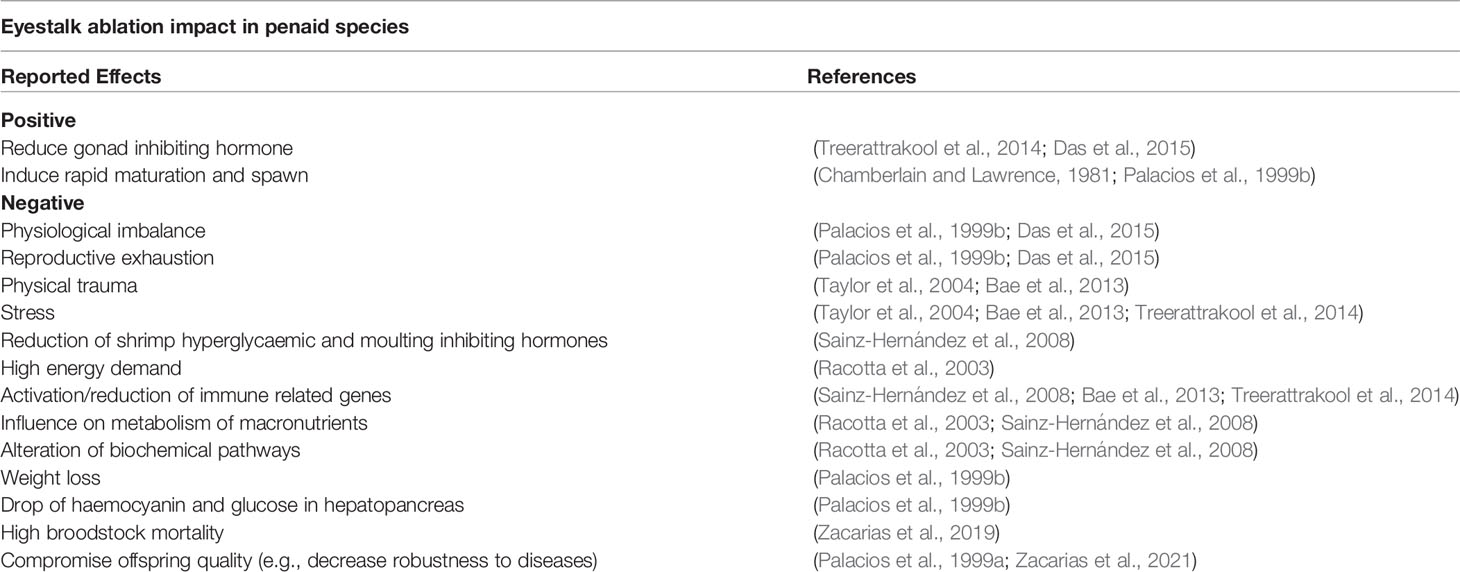
Table 1 Summary of eyestalk ablation effect in Penaeids (includes data from P. vannamei, P. monodon and P. stylirostris).
Due to the multiple negative effects associated with ablation (Table 1) and concerning the welfare of P. vannamei broodstock, predictable maturation and spawning of captive specimens without the use of eyestalk ablation has been considered as a long-term goal for the industry (Quackenbush, 2015). Recent reports have suggested that similar productivity in broodstock can be obtained without eyestalk ablation through the application of husbandry interventions, including pre-maturation conditioning, increased stocking density and/or altered sex ratios (Zacarias et al., 2019). Trials conducted using these practices have demonstrated that rapid maturation and re-maturation of non-ablated P. vannamei females can be obtained while maintaining similar production levels of eggs/nauplii compared to ablated females (Zacarias et al., 2019; Zacarias et al., 2021). In addition, genetic background has been suggested to play a role in the production without eyestalk ablation. Therefore, ablation of eyestalks may no longer be required, which would represent an important step towards improved animal welfare. As an example, there are a few regular maturation facilities in Central and Latin America (Brazil, Colombia, Ecuador, and México), and Asia (Thailand) that are no longer using eyestalk ablation (Patrick Sorgeloos, 2020. Pers. Comm; Robsons McIntosh, 2020. Pers. Comm.). To support the phase-out of such practice, further studies to quantify the trade-offs from both production and economic perspectives would be very useful for the industry.
2.2 Disease and Stress-Immunity Mechanisms in Farmed Tropical Penaeids
Disease remains the key limiting factor for sustainable food production from crustacean aquaculture and fishing efforts. At the time of writing, 10 out of 11 diseases listed for crustaceans by the World Organisation for Animal Health1 blight penaeid shrimp aquaculture (listed in Table 2). Viral (IHHNV, TSV, WSSV, YHV) pathogens dominated a series of debilitating disease outbreaks across Asia in the 1980s and 1990s, whereas bacterial (Vibrio parahaemolyticus) and fungal (Enterocytozoon hepatopenaei) pathogens emerged in the 2000s and 2010s (Thitamadee et al., 2016; Shinn et al., 2018; de Souza Valente and Wan, 2021). Substantive financial losses are incurred due to high mortality rates, up to 100% within days-weeks in many cases, and shrimp that survive are usually stunted and deformed, leading to high levels of waste (some features are listed in Table 2).
2.2.1 Biological Defences
To prevent, and manage, the spread of disease in decapod aquaculture, extensive efforts have been made to characterise their biological defences (Bachère, 2000; Tassanakajon et al., 2018; Gong and Zhang, 2021). Simply, innate immunity is split across three categories of defence (Figure 1): physical barriers (1), cell-directed responses carried out by haemocytes (2), and humoral factors produced by a variety of tissues that recognise, immobilise, and kill pathogens (3). What follows is a synopsis of the major features of decapod innate immunity – the articles cited provide more detail of these topics.
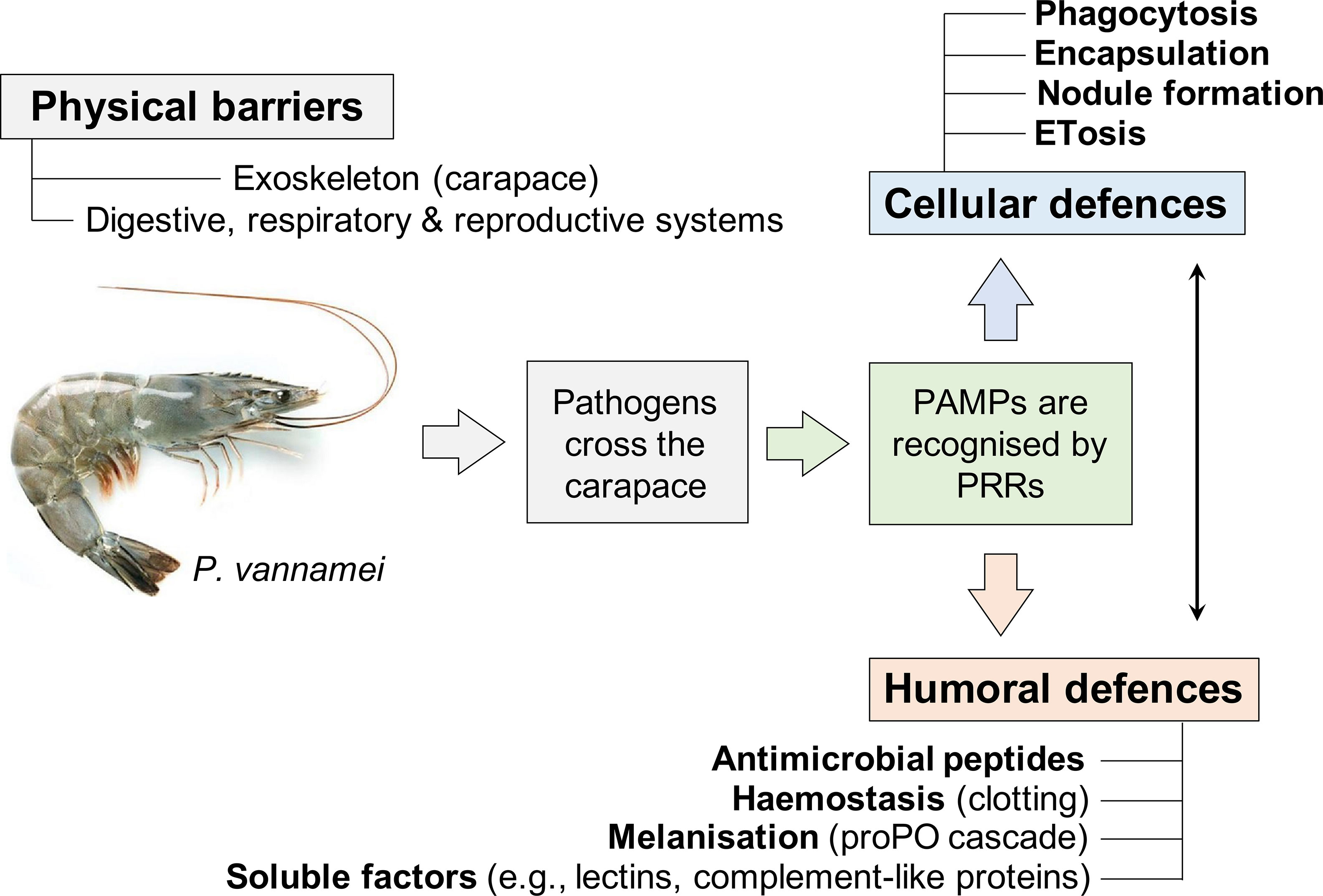
Figure 1 Innate immune defences of penaeid shrimp to infectious disease. Should the disease-causing agents circumvent the physico-chemical barriers, their ligands (pathogen associated molecular patterns, PAMPs) are intercepted by pathogen recognition receptors (PRRs) freely dissolved in the haemolymph or located at the surface of haemocyte plasma membranes. Such recognition triggers a multitude of immune-related cascades including the ingestion of pathogens (phagocytosis), the ensheathment of pathogens – nodules for numerous microparasites, and capsules for larger parasites – and the release of chromatin extracellular traps (ETosis). Bacteria, fungi, and viruses are targeted directly by a series of microbiostatic and microbicidal factors (e.g., lysozyme, lectins) and indirectly by toxic by-products and intermediates of melanogenesis (via the activation of the proPhenoloxidase (proPO) cascade). There is much crosstalk between the cellular and humoral defences (indicated by the black arrow), such as, haemolymph gelation/clotting.
Physical (1). Disease-causing agents need to enter a host for survival, to replicate, and occasionally, complete their lifecycle. The penaeid carapace represents an important biological barrier – cuticle layers formed with chitinous polymers are hardened through the addition of calcium salts (external surface > epicuticle > exocuticle > endocuticle > epidermis > haemocoel > organs). The exoskeleton restricts most pathogens – some fungi and oomycetes are exceptions – to a few sites of entry (Coates et al., 2022); the gastrointestinal, reproductive, and respiratory systems as well as the nephrocomplex (i.e., antennal gland) (De Gryse et al., 2020; Liu et al., 2021). For example, Qi et al. (2017) highlighted the importance of the intestinal barrier in shrimp-vibrio antibiosis. The authors interrogated the intestinal transcriptome of P. vannamei infected with the causative agent of acute hepatopancreatic necrosis syndrome (AHPNS), namely V. parahaemolyticus, to reveal ~2,500 upregulated genes with functions in immunity and cellular restructuring, e.g., pathogen recognition, phenoloxidase activity, antimicrobial peptides.
Cellular (2). Should the physiochemical barriers fail, a heterogenous population of circulating immune cells termed haemocytes (granular, semi-granular and hyaline cells), can intercept the pathogenic intruders, reviewed by Johansson et al. (2000). Crustacean haemocytes perform tissue surveillance and recognise ‘non-self’ carbohydrate-lipid complexes that coat the surfaces of microbes; β-glucans and mannans for fungi (Vargas-Albores and Yepiz-Plascencia, 2000; Yepiz-Plascencia et al., 2000), lipopolysaccharides (endotoxins) and lipoteichoic acids for Gram-negative and Gram-positive bacteria (Phupet et al., 2018), respectively. Depending on the size of the pathogen (micro vs macro), the numbers of intruders present and the target tissue, haemocytes respond in several well-defined ways (Figure 1).
● Phagocytosis – direct ingestion of the microbial target into the cytoplasm and subsequent destruction via lysis facilitated mostly by enzymes, reviewed by Liu et al. (2020).
● Degranulation – the release (extra-cellularisation) of cytoplasm-derived granules containing a concoction of anti-infective factors, including antimicrobial peptides, adhesives, and the components of the phenoloxidase cascade (Smith and Söderhäll, 1983). Degranulation precedes nodule/capsule formation.
● Nodule formation – cellular aggregates consisting of numerous microbes (usually bacteria or yeast-like cells), host haemocytes, and melanotic debris. Such nodules are usually sequestered to specific tissues like the hepatopancreas and gills (Smith and Ratcliffe, 1980; Davies et al., 2020).
● Encapsulation – the ‘walling off’ or ‘ensheathment’ of microbes, microeukaryotes, parasitoids (too large to phagocytose), and damaged host tissue in recurrent layers of haemocytes. After multiple layers of haemocytes are in place, melanin is used to coat the capsule (van de Braak et al., 2002; Costa et al., 2009).
● Extracellular trap release (ETosis) – discharge of decondensed chromatin embedded with proteinaceous factors. Microbes are caught in the ‘chromatin net’ and are vulnerable to multiple biocidal agents, including histone-derived peptides and peroxidase (by)products (Ng et al., 2013; Robb et al., 2014).
Humoral (3). There is much coordination and crosstalk between the so called cellular and humoral immune reactions in decapods – wound repair, clot formation (haemostasis) and pro-phenoloxidase activation being key examples (Fontaine and Lightner, 1973; Söderhäll and Smith, 1983; Maningas et al., 2013). A myriad of enzymatic and non-enzymatic factors, including agglutinins, lysins, cytokine-like molecules and antimicrobial peptides (e.g., crustins; Bartlett et al., 2002), are found in the haemolymph of decapods. Many are derived from the haemocytes (e.g., clotting pathway components), while others are released constitutively by diverse tissue types including the hepatopancreas, with the remainder being acute phase (Smith and Chisholm, 1992; Suleiman et al., 2017; Tassanakajon et al., 2018). Arguably, the most identifiable response is the generation of the black/brown pigment melanin from phenoloxidase activities. Melanin itself is microbiostatic and virustatic, whereas the by-products generated from its biogenesis (e.g., oxygenic radicals), are highly toxic (Cerenius et al., 2010; Coates and Talbot, 2018). Multiple enzymes – laccase, tyrosinase, catecholoxidase and haemocyanin – are capable of catalysing simple phenols into quinones, which go on to form melanin (Coates and Costa-Paiva, 2020). These enzymes are usually referred to collectively as phenoloxidases and display multiple immune properties (Cerenius and Söderhäll, 2021).
2.3 Environmental and Biological Conditions During Grow-Out: Health-Stress-Disease Axis in Ponds
The health of farmed fish is inextricably linked to their welfare (Segner et al., 2012), and unsurprisingly, this is also the case for decapod crustaceans such as penaeid shrimps, as illustrated by the Disease triangle in Figure 2. There remains a deficit with respect to welfare indicators or standardised reference intervals for monitoring penaeids in captivity, partly because it is challenging to define what constitutes a healthy shrimp (Coates and Söderhäll, 2021). Recent efforts have looked to the gut microbiome as a means of measuring ‘healthy’ penaeids, including immune vigour (Li et al., 2018; Holt et al., 2021). Unsurprisingly, the resident microbes of penaeid tissues are linked to disease and health outcomes – the composition, diversity, and balance between pathobionts and symbionts.
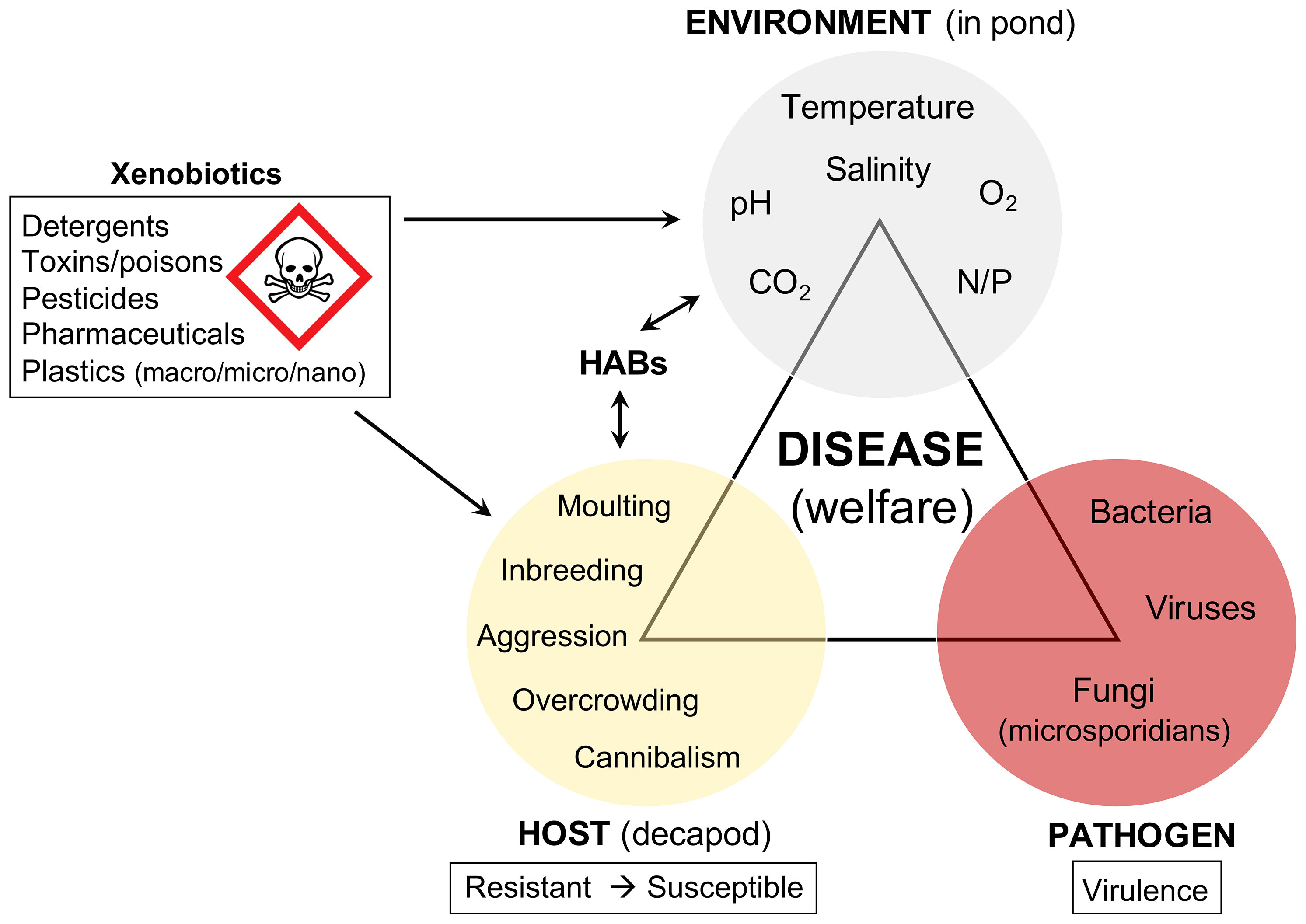
Figure 2 Disease triangle in the context of penaeid welfare (in pond). Growth and maintenance of shrimp under culture conditions poses several challenges for shrimp health and welfare. Susceptible hosts, combined with virulent pathogens and unfavourable environmental conditions encourage disease outbreaks. The presence of immune-modulating xenobiotics, fluctuating abiotic factors leading to hypoxia, hypercapnia, or acidosis can tip the balance in favour of the pathogen(s). HABs, harmful algal blooms; N, nitrogen; P, phosphate.
Acute, subacute, prolonged, and chronic perturbations in pond abiotic factors – temperature (Cheng et al., 2005; Rahman et al., 2006), pH (Gunalan et al., 2010), salinity (Li et al., 2008), nitrites (Tseng and Chen, 2004), and hypoxia/hypercapnia (Burgents et al., 2005) – influence decapod susceptibility to disease. Acute stress can stimulate the immune system and tends to have transient impacts on the host, sometimes promoting stress tolerance to subsequent exposure. Prolonged stress, from one or more source (chemical versus physical) can lead to shifts in metabolism, immune dysfunction, and health declines. Imbalances of dissolved oxygen and carbon dioxide in the water (hypoxia, and hypercapnia, respectively) can lead to tissue dysoxia and lactate accumulation – the switch to suboptimal glycolytic metabolism over oxidative phosphorylation. Temperature, pH and dissolved gas content are inextricably linked. As penaeids are ectotherms, temperature extremes outside of their tolerance range can have profound effects on general physiology. Indirectly, higher water temperatures retain less O2 and increased pCO2 in the water leads to acidosis. CO2 can also bind with H2O in the haemolymph to form H2CO3 (carbonic acid) (reviewed by Coates and Söderhäll, 2021; Millard et al., 2021).
From a biological perspective, one of the key questions for both welfare and production is the effects of stocking density. For this reason, there are numerous studies that have assessed the effects of stocking density on P. vannamei at different life stages (Froes et al., 2013; Wasielesky et al., 2013; Esparza-Leal et al., 2015) and under different production systems (Cuvin-Aralar et al., 2009; Wasielesky et al., 2013; Durairaj et al., 2018). While most of these studies have concentrated on the impacts from a production perspective there are some studies available on the effects of stocking density on the behaviour of P. vannamei (da Costa et al., 2016; Bardera et al., 2021). Results from (da Costa et al., 2016) showed that under aquaria conditions stocking density affects the behaviour of P. vannamei juveniles and that there is an optimum animal density that promotes behaviours such as ‘searching’ and ‘feeding’. Dominance hierarchies have been reported and results revealed that at least at the juvenile stage, higher densities (around 25 juveniles m-2 compared to 6 and 12 juveniles m-2) maximise feeding behaviour while minimising the effects of dominance. However, the translation of such observations to real productions systems is challenging and therefore studies on real production systems are mainly based on production outputs. In this sense, the effect of stocking density in relation to temperature has been modelled and data indicate the importance of tailoring stocking density based on the production system and temperature as even minor differences in temperature can produce significant differences in growth and therein profits (Villanueva et al., 2013; Araneda et al., 2020).
In summary, it is difficult to recommend unique values for the main biological factors as they are extremely dependent on the life stage, the actual production system (sometimes associated with different regions) and the environmental conditions the animals experience. However, standardised farming management practices (including animal handling), stable pond/tank conditions (e.g. water quality parameters remaining in ideal range, enough space for shrimp to grow, presence of natural diet and beneficial microbial communities), and routine health checks are necessary to avoid stress, fatalities and financial loss.
3 Welfare Indicators in Decapod Crustaceans
3.1 Current State on Physiological Biomarkers
In decapod crustaceans, as in vertebrates, disturbances in the nutritional, environmental and health welfare domains can lead to allostatic overload (Freire et al., 2020). Allostasis, defined as the adaptive process for actively maintaining stability through change (Sterling and Eyer, 1988) is relevant when trying to establish reference physiological intervals. In this sense, physiological responses that can be measured and that are associated with homeostasis and allostasis could be relevant as direct welfare indicators. The concept of using physiological measures as welfare indicators is particularly relevant for aquatic species where assessing behaviour, pain and suffering is not easy or not possible due to lack of knowledge (Jerez-Cepa and Ruiz-Jarabo, 2021). For these species, the physiological framework is based on the fact that a number of physiological parameters will move outside their allostatic range (overload). These changes must be associated with the different types and degree of stress that the animals experience. Decapod species, like any other organism, respond to stressors by mounting a stress response that involves behavioural and physiological changes at multiple biological levels. The concept of finding suitable biomarkers that can be applied in the context of decapod welfare has been mentioned in few studies (Rosas et al., 2004; Ciaramella et al., 2014; D’Agaro et al., 2014; Wang et al., 2014; Albalat et al., 2019). However, there is little evidence that a concerted effort has been made to establish haematologic biomarkers or reference intervals for decapods, more specifically Penaeus spp., in captivity or under cultivation. Tu et al. (2010) trialled some measures – e.g., activities of glutathione-S-transferase, peroxidase and catalase – in P. monodon production sites along the Mekong River Delta (Vietnam) and could discriminate between animals reared in intensive systems versus those reared in integrated systems. Producing a suite of biomarkers for decapods is not straightforward as routine measures like cell (haemocyte) counts, protein levels or haemolymph enzyme activity (e.g., lysozyme, phenoloxidase) can fluctuate according to season (Hauton et al., 1997) moult cycle (inter-moult, pre-moult, post-moult; Liu et al., 2004) or even lunar phases (Bautista-Covarrubias et al., 2020).
Rodríguez and Le Moullac (2000) reviewed health control measures of penaeid shrimp and cited 120 mg [protein] mL-1 [haemolymph] for laboratory reared P. vannamei juveniles. Perhaps some inspiration can be taken from a marine invertebrate often misidentified as a decapod, the Atlantic horseshoe crab Limulus polyphemus. Horseshoe crabs are highly valued due to the sensitive endotoxin detection kit (Limulus Amebocyte Lysate) derived from the cellular fraction of their blood (haemolymph), which replaces the rabbit pyrogen test in pharmaceutical and vaccine development (Krisfalusi-Gannon et al., 2018). Because of this commercial sensitivity, reference intervals have been proposed for captive L. polyphemus ex situ (Smith et al., 2002) and free-ranging crabs in situ (Arnold et al., 2021). As horseshoe crabs suffer from a protein deficiency syndrome in captivity (Smith and Berkson, 2005), an acceptable range is considered ~34 to 112 mg [protein] mL-1 [haemolymph] – with most of the soluble protein made up of haemocyanin (>90%; Coates et al., 2012). Haemocyanin comprises >60% of haemolymph protein in most decapods studied to date (e.g., Nephrops norvegicus, Coates and Nairn, 2013). Among the numerous cellular and biochemical indicators proposed across the literature, total protein levels and haemocyte counts represent the most likely candidates, in addition to lactate levels, as ‘welfare’ or ‘health’ indicators for decapods. These procedures are invasive, but not lethal, in the right hands. Some such measures have been used in an attempt to disentangle ‘captivity-associated stress’ in crabs (e.g., Cancer pagurus; Johnson et al., 2016) and lobsters (e.g., Homarus gammarus, Basti et al., 2010; Albalat et al., 2019). Haemolymph biochemical parameter assessment has been trialled with autoanalyzers (haemocytes) and handheld refractometers, e.g., crayfish (Astacus leptodactylus) and lobsters (H. americanus) (Sepici-Dinçel et al., 2013; Wang and McGaw, 2014).
A condition/welfare index based on both physical appearance and behaviour (e.g. ‘vigour’) as developed by Albalat et al. (2017) for trawl-caught langoustines N. norvegicus, combined with one or more haemolymph measures, would represent a major step forward.
3.2 Operational Welfare Indicators (OWIs): Individual and Group Based, Direct and Indirect and Invasive vs. Non-Invasive
If farmers want to detect welfare problems during the farming of crustaceans, they must have good feasible operational welfare indicators (OWIs) that can be measured quantitatively by staff based at the farms. OWIs must be species- and life stage-specific, minimally invasive, fit for purpose (for production systems and operations) and robust. In Table 3, we suggest a list of positive and negative survival and situation-related factors to be observed based on the 5 domains framework suggested by Mellor and Reid (1994) and based on animal (outcomes) and resource (inputs) measures applicable to the crustaceans welfare.
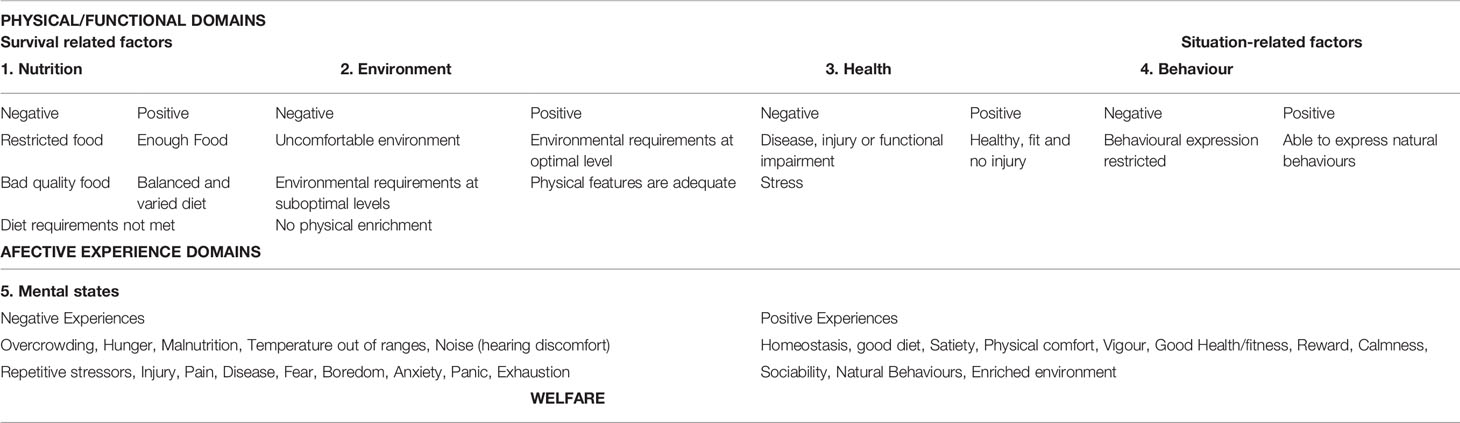
Table 3 List of negative and positive survival and situation related factors to be observed in order to generate the best Operational Welfare Indicators (OWIs) for the species based on the 5 domains framework (adapted from Mellor and Reid, 1994 to crustaceans).
Each of the risk factors and stressors during the life cycle of the farmed crustaceans can be assessed by a welfare indicator closely related to the original stressor. For example, acute or unexpected changes in water temperature (the stressor) can lead to changes of the appetite level in the farmed crustaceans. Monitoring and evaluation of the feeding responses is a common OWI potentially related to an environmental stressor (Martins et al., 2012). Real time monitoring of the animals and the environmental parameters is key for the identification of environmental-related welfare issues.
In order to be able to measure the different welfare domains, we present in Table 4 a list of potential direct (animal) and indirect (environment-based) welfare indicators for P. vannamei, that could also be used for other decapod crustaceans as appropriate. Direct individual based OWIs include both physical health and physiological parameters as well as behavioural measures. On the other hand, indirect or resource-based measures include environmental parameters related to water quality, husbandry procedures, type of food and other measures such as predator presence or enclosure design and substrate access (e.g. environmental enrichment such as the background colour of tanks, use of hides and substrates or different feeding methods).
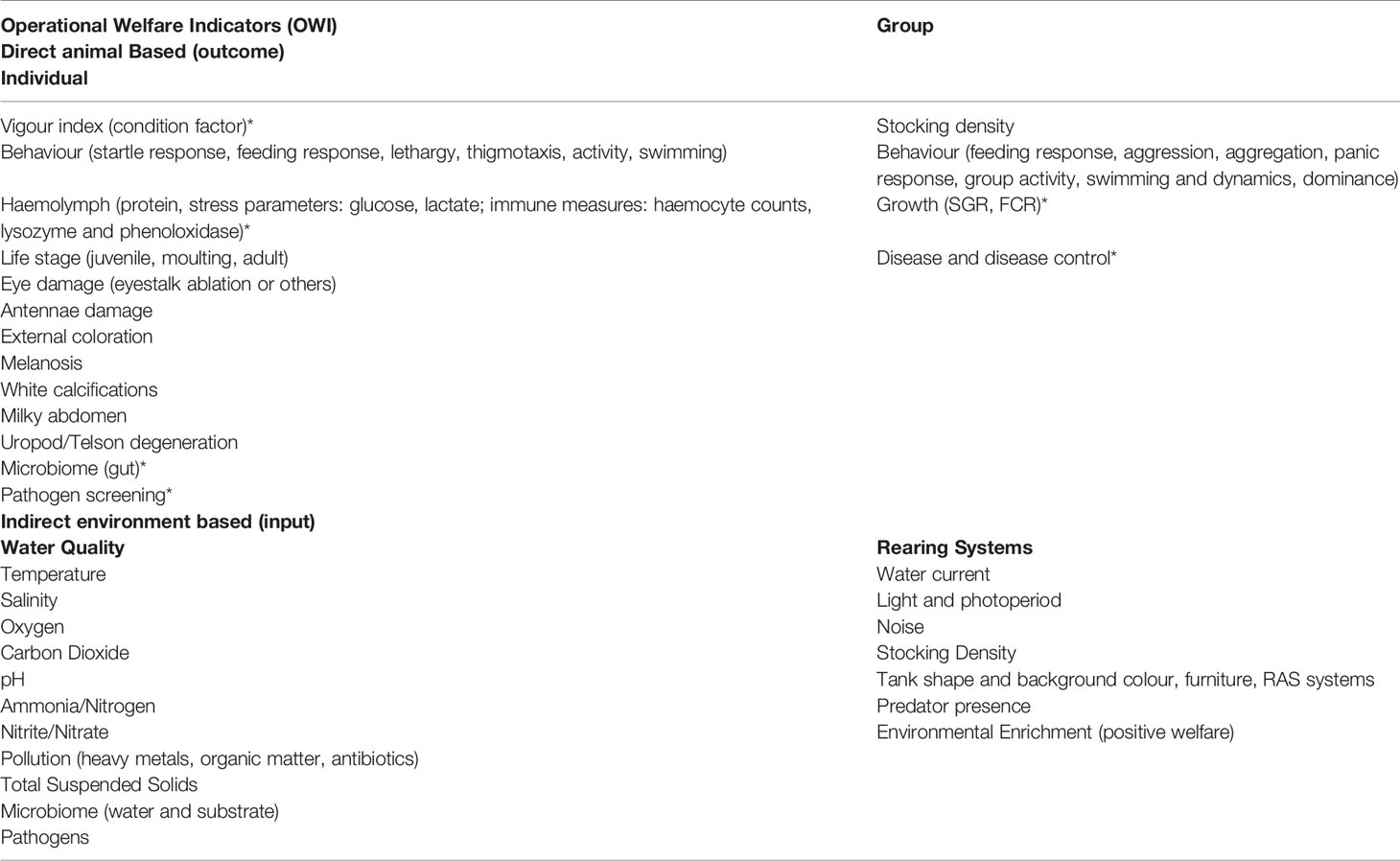
Table 4 List of potential OWIs to be measured for welfare assessment and their use as a decision support method. Invasive OWIs are highlighted using an asterisk (*).
OWIs must be used at different levels depending on the intensity of the intervention needed (Noble et al., 2018). OWIs at the primary level of assessment include environmental parameters, visual observations (behaviour and appearance) and sudden changes in relation to them. When this is not sufficient to evaluate the welfare status of the animals then it is necessary to move to a secondary level of assessment and use the more invasive handling and laboratory-based OWIs (indicated in orange in Table 4) that require physical manipulation of the animals (vigour index or close visual assessment of the damage or disease). If the welfare problem is not solved then the next stage would be the tertiary level of assessment where handling and sampling of blood and tissues is required (anaesthetics are recommended for this sampling procedure) with more detailed analysis need to be performed: PCR for disease diagnosis, histopathology, haemolymph sampling for stress parameters or other techniques might be required: behavioural assessment under controlled conditions, etc.
3.3 Other Emerging Operational Welfare Indicators
Biosensors, loggers and early warning systems are: cheap, user-friendly, non-invasive methods for early detection of critical alterations in the farming conditions. They can be automatic systems for behavioral observations or water quality sensors (Barreto et al., 2022). With the use of Artificial Intelligence (AI) and machine learning methods the monitoring of environmental parameters and behaviour can be done automatically, and the decision-making process becomes more accurate (O'Donncha et al., 2021) and autonomous. These methods of precision farming (PF) are being developed for finfish and molluscs with proven success. In shrimp farming, there are examples of monitoring systems to automatically record changes in physicochemical water parameters that are processed using AI (Borquez-Lopez et al., 2016). However, AI models could, for example, identify some of the animal-based OWIs in P. vannamei, such as body melanosis, antenna and eye damage, and could also monitor their behaviour and assess possible risk factors, based on individual and group behaviour, interaction with environment and social behaviour (aggression, aggregation, feeding responses, etc) (Huang et al., 2019).
Use of rapid on-site testing for disease diagnostics is another emerging health and welfare tool. In general, in aquaculture systems, animal mortality results from complex interactions between genetic background, environmental conditions, stress and opportunistic pathogens, making most laboratory approaches sub-optimal. The on-site testing makes it possible to obtain physiological and behavioural responses to the real environmental conditions and to assess their welfare in relation to those responses (Barreto et al., 2022). Some of those on-site diagnostics are cheap and affordable paper-based diagnostics, widely used for human health (Hu et al., 2014) and recently translated into fish farming2. Other advances in welfare indicators are the development of smartphone based diagnostic technologies (Rateni et al., 2017) as well as smartphone applications for visual based diagnostics and the use of cloud metadata3.
The importance of promoting positive welfare and the need to offer more natural environments for the cultured animals has increased in recent years. The use of environmental enrichment (EE) to increase the complexity on the environment e.g., hiding sites, tanks of various colours and shapes, flows and natural light regimes, life feeding or feeding methods that mimic their natural behaviours, are crucial to promote social interaction, to avoid maladaptive behaviours and to reduce stress related to captive conditions (Arechavala-Lopez et al., 2021). In decapod crustaceans, as observed in fish and other farmed vertebrates, the use of EE could be used to improve their welfare status. EE could be developed on temperature gradients (behavioural prophylaxis) based on thermal preferences and related to their internal emotional states (Rey et al., 2015). Other structural EE are already used for P. vannamei such as a dark background colour of the tanks or the rounded shape has been mentioned before.
3.4 Existing Aquaculture Standards Applied to the Farming Practices of P. vannamei: Gaps in Welfare Indicators
For the farming of decapod crustaceans and specifically for penaeid shrimp farming the key factors that need to be identified and if necessary revised include feed quality and delivery methods, water quality and flow, stocking density, transport methods and the number of transport events during shrimps’ life cycle. The main management practices and interventions to be considered include alternatives to eyestalk ablation for broodstock, grading of juveniles and disease treatments up to point of slaughter. In this way, a good welfare assessment can be achieved for each of the potential stressors, leading to improved husbandry practices.
Most commercial shrimp aquaculture standards use different welfare indicators related to the environment and the health of the animals (input and outcome measures). Few use the 5th domain on the mental status of the animals that should be the main factor to determine the outcome of the welfare status of the animals (Table 3).
The Aquaculture Stewardship Council (ASC) Shrimp Standard4, for example, places a lot of emphasis on the effect of shrimp farming on biodiversity and impacts on the environment, as well as the use of wild fish as an ingredient for feed. Farms are required to measure water quality parameters, as well as, monitor diseases and report during auditing. The use of antibiotics is restricted, and they should be monitored in the water systems. Social aspects of the farming practices are also included. However, no direct animal-based welfare measures and targets are included besides from the routine health checks. No morphological, physiological or behavioural OWIs are required.
Other standards such as the Asian Seafood Improvement Collaborative5 (ASIC) shrimp standard or the Best Aquaculture Practice6 (BAP) standards for finfish and crustacean farms are based on best management practices with very general considerations regarding the use of OWIs (stocking densities, feeding, fasting, crowding, and dewatering, water quality, behaviour and condition and testing for disease outbreaks). Humane slaughter techniques are mentioned but with no specifics given. The main issues addressed are environmental, social and those concerning food safety (drug and chemical management, microbial sanitation, hygiene, harvest and transport, biosecurity and traceability). ASIC requirements for shrimp health status are limited to compliance to existing national standards and the stocking densities need to be recorded to monitor and estimate the number of escapes. Mortalities need to be recorded, as well as, the main environmental parameters (temperature, DO, salinity and ammonia). BAP contains a short section on shrimp-specific standards related also to environmental and effluent management (water exchange), as well as, to food safety during harvest and transport (e.g. the use of sulphite solutions as antimelanotic at harvest). It also specifies a maximum biomass limit based on performance measures for health and survival. BAP requires training for staff to provide appropriate levels of husbandry and awareness of these standards.
In Asia, there are also local government standards that address some health and welfare issues, and also focus on animal welfare indicators7. These standards contain specific information on best practices and the recommended range of stocking densities for the different culture systems. However, the recommendations on animal welfare are still very general, with no specific animal-based OWIs required.
Therefore, most common shrimp standards are still using very few animal-based OWIs and recommendations are very general, with no guidance on ranges of tolerance or rates for the most common parameters provided. For example, standards should provide guidance on the rates of survival or mortality rates per life stages of shrimp production. Feeding should be focused on nutritional requirements and best feeding practices. Maximum fasting times should be provided, in addition to guidance on crowding prevention techniques and disease prevention and monitoring of relevant parameters. Regulation on humane stunning and slaughter methods and the use of drugs and chemicals, as well as handling standards should be provided. Most of those parameters have been studied and there is sufficient knowledge in the scientific literature to be used in farming protocols and standards. Our review is an example of the amount of knowledge that exists on shrimp farming and mitigation methods.
4 Stunning and Slaughter Practices in Relation to Sentience
4.1 Current Scientific Evidence for Sentience in Decapod Crustaceans
Sentience can be defined as having the capacity to feel (Kirkwood, 2006) or following Darwin’s definition ‘a sentient animal is one for whom feelings matter’ (Darwin, 1872). Within the context of this review, we will more specifically align with the Animal Welfare Committee in the UK which has defined sentience as the capacity to experience pain, distress or harm, although as pointed out by other authors this definition lacks the consideration of animals being able to experience positive feelings (Mellor and Beausoleil, 2015; Birch et al., 2021). In any case, the capacity for a living organism to be capable to have feelings such as pain requires a level of awareness and cognitive ability (Broom, 2019). So far, arguments in support of the view that decapod crustaceans have the capacity to feel pain have been made by some authors (Conte et al., 2021; Passantino et al., 2021) while others support the view that the evidence is currently insufficient (Diggles, 2019).
Frameworks to provide scientific evidence of pain in vertebrate species have been proposed and generally agreed for several years (Bateson, 1991). The translation of such frameworks seeking to establish if invertebrates experience pain has been presented by a number of authors (Smith, 1991; Smith and Boyd, 1991; Birch et al., 2021) (Table 5).
Upon examining research performed in P. vannamei, current evidence of sentience in decapod crustaceans is mainly concentrated in what it is known for the largest decapods (crabs, lobsters and crayfish), which do not include penaid species. From a ‘neurological perspective’, three neutropil areas (terminal medullae, hemiellipsoid bodies and the accessory lobes) are candidates for higher integrative centers (Sandeman and Harzsch, 2016). The terminal medullae and the hemiellipsoid bodies are part of the lateral protocerebrum. Recent research on decapod crustacean brains (supraesophageal ganglia) (Strausfeld et al., 2020) indicate the presence of protocerebral centers in P. vannamei. Immunohistochemistry results showed the presence of a substantial lateral protocerebral and optic neuropils and a much reduced anti-DC0-immunereactive centre, the location of which corresponds to the ancestral mushroom body of Stomatopoda and Caridea. This area, therefore, comprises an integrative centre that is associated with the hemiellipsoid body (homologous to insect mushrooms bodies) linked to learning and memory.
From a ‘cognitive and behavioural perspective’, the little research on P. vannamei published to date has mainly focused on feeding behaviour, production practices such as stocking densities and mating behaviour (Yano et al., 1988; Bardera et al., 2020; Bardera et al., 2021). A review by Bardera et al. (2019) highlighted the importance of studying behaviour for the farming/production of P. vannamei, but studies on motivational trade-offs, flexible self-protective behaviours in response to injury, threat and associative learning that goes beyond habituation and sensitisation are currently missing. In this regard, recent studies performed in understanding agonistic behaviours in the cultured swimming crab (Portunus trituberculatus) (e.g. Su et al., 2021; Wu et al., 2021) and the interaction between animal behaviour and production practices (e.g. Zhang et al., 2021) highlight the importance to factor behavioural aspects in production systems for decapod species.
Finally, from a ‘mixed perspective’, Taylor et al. (2004) examined the role of a topical anaesthetic (lidocaine) and a coagulation agent in minimising the stress response due to eyestalk ablation in P. vannamei. Their results indicate that the application of an anaesthetic significantly improves the elapsed time of feeding onset and their swimming behaviour. On the other hand, the effects of anaesthetics (clove oil/eugenol) have also been examined in a number of decapods, including P. vannamei (Parodi et al., 2012; Wycoff et al., 2018; Becker et al., 2021). Results from Wycoff et al. (2018) show that P. vannamei was very susceptible to stress and therefore measurements of heart rate, motor nerve activity and sensory rate activity were inconclusive or not possible to perform. However, the study showed that the animals were lethargic within 2 min, and they were inactive to tail pinches within 6 to 12 min when bathed at a eugenol concentration of 200 ppm.
As the number of studies is certainly limited, especially from a cognitive and behavioural perspective it is clear that more fundamental research in this area is needed. However, following the ‘precautionary principle’ the basic requirement to define current production practices from an animal welfare perspective is relevant. In the case of P. vannamei, the demand for increased welfare could potentially be driven not only by stakeholders and certification schemes but also by consumers based in key importing countries, since a very significant proportion of these animals are traded internationally. Therefore, in the next section we evaluate key challenges associated with the stunning and slaughter of P. vannamei from an animal welfare perspective.
4.2 Stunning and Slaughter Practices in P. vannamei
Partly due to the current lack of welfare protection for decapod crustaceans (see Current Legislation Relevant to Decapod Crustaceans); harvesting, stunning and slaughter practices for this group in general, and P. vannamei in particular, are not regulated, or even standardised. Before entering into further detail, it might be relevant to indicate that within the context of this review we have used the terminology of Conte et al. (2021) whereby ‘stunning’ refers to rendering the animals insensible, and that this process can be reversible or irreversible (in the latter case it should be considered as ‘slaughter’). Methods for stunning and slaughter used in decapod crustaceans are varied and include cold shock in air, ice or slurry ice, boiling, freshwater or salt baths, carbon dioxide narcosis, high pressure, mechanical splitting or spiking of the nervous system, dismemberment and electrical stunning as reviewed by Conte et al. (2021). The variety of techniques used not only reflects the absence of legislation but also the fact that decapod species are very diverse in their anatomy and physiology. Decapod crustaceans are held and slaughtered for different ends, e.g., scientific research studies versus food production, and in the latter case, there may be the additional issue of dealing with very large numbers of animals, which is true for P. vannamei.
A requirement to use more humane methods of slaughter is now demanded by advocacy bodies, increasingly being mandated by legislative bodies and governments in several parts of the world, and is currently being debated in the UK (see Current Legislation Relevant to Decapod Crustaceans). When applied to decapod crustaceans, the majority of methods mentioned above are considered inhumane by the European Union’s Scientific Panel on Animal Health and Welfare (Animal Health and Animal Welfare Panel of the European Food Standards Agency, 2005) since most take some time to have an effect and hence have the potential to confer suffering or stress. Methods of rapid dispatch that minimise the potential to inflict suffering and stress, and thus improve welfare, continue to be sought by the crustacean fishery and aquaculture industries. Currently, there is no published information on the commercial slaughter practices for penaeid shrimps, and for that reason it is difficult to ascertain if these are applied correctly to minimise peri-slaughter stress of P. vannamei during the harvest process.
A previous study has shown that the use of slurry ice on P. vannamei (thought to be the most commonly used slaughter method) renders the animals sedated, accompanied by a reduced cardiac function within seconds (substantial reduction within 30 seconds) (Weineck et al., 2018). Despite a decrease in heart rate, specimens would tail flip within 1 min when exposed to cold water and more importantly, upon transfer to warm water the heart rate increased, indicating that the stunning is reversible. Therefore, although slurry ice proved to be a fast method for stunning, controlling the time and conditions between stunning and slaughter in commercial farms would be very important from a welfare perspective. Regarding electrical stunning, several references are available for the larger decapods (Neil, 2010; Roth and Øines, 2010; Neil, 2012a; Roth and Grimsbø, 2013; Fregin and Bickmeyer, 2016; Roth and Grimsbø, 2016). These studies have shown the effectiveness of electrostunning in rendering these larger decapods insensible rapidly (within 1 second reported) (Roth and Øines, 2010). The effectiveness of electrical stunning in P. vannamei was also evaluated recently (Weineck et al., 2018), and was found to be as effective, but less consistent than cold stun in ice slurry.
The choice of slaughter method will also impact post-harvest product quality (Neil, 2012b), and a study performed on P. vannamei has shown that the use of slurry ice is more beneficial for post-harvest quality than cold storage and flake ice (Wang et al., 2014). Inappropriate harvesting, stunning and slaughter trigger an acute stress response, which can impact the initial post-mortem metabolism and reduce product freshness (Albalat et al., 2009; Gornik et al., 2010). Furthermore, other stress-related consequences such as extensive autotomy (the casting off a part of the body by an animal under threat) has been reported when crabs were stunned using a low electrical field strength to the whole animal (Roth and Øines, 2010) an effect not reported with higher electrical field strengths (Neil, 2010; Neil, 2012a). Blackening or melanosis development can also be affected if the stunning/slaughter method causes an increase in temperature (Albalat et al., 2022).
5 Current Legislation Relevant to Decapod Crustaceans
Countries that protect decapods or place them under some degree of legal protection are Australia, Austria, New Zealand and Norway. Italy and UK do have some partial protection or changes in legislations are being contemplated (in UK).
Australia: In 2004, Australia adopted the Australian Animal Welfare Strategy (AAWS), which explicitly covered ‘all sentient animals—that is, those with a capacity to experience suffering and pleasure’. Crustaceans are only protected in some states and by some provisions. These provisions cover cephalopods (Australian Capital Territory) and crustaceans (Australian Capital Territory and New South Wales – for human consumption; Victoria – adult decapods since 1997). However, there is room for improvement in many domains related to animal welfare in general, Australia ranking D on the animal protection index8. Animal sentience is still not recognised at the Commonwealth level.
Austria: The Austrian Animal Welfare act of 20049 protects crustaceans under national husbandry guidelines. It has been scored as B on the animal protection index10.
Switzerland: The Animal Welfare Ordinance from 200811 protects crustaceans during slaughter and during transport (stunning is required and no ice is allowed during transport)
New Zealand: Animal Welfare act in 199912 changed the definition of the NZ animal animal protection act (1960) to cover crabs, lobsters and crayfish.
Norway: Legal protection for decapods by the Norwegian Animal Welfare Act (2010)13 including killing, confining and transport.
The UK government commissioned a report in 2021 to evaluate the current evidence on the welfare status of crustaceans and the potential for them to be sentient (Birch et al., 2021). Following the report DEFRA tabled an amendment to the Animal Welfare (Sentience) Bill was approved in April 2022 and now includes decapod crustaceans and cephalopod molluscs.
Other countries such as Italy have partial protection in some provinces/regions and since 2017 crustaceans cannot be kept on ice in restaurant kitchens (all regions) or boiled alive (in the province of Regno Emilia for example).
6 Concluding Remarks
Despite the wide knowledge on crustacean nutritional requirements, stress physiology and immunology as well as disease control, still little is known about some key parameters related to the five welfare dimensions, as they might be applied to penaeid shrimp, and some of these gaps have been identified in this review. The main challenges facing the aquaculture of penaeid shrimp such as P. vannamei today are still based on improving best management practices (BMPs) taking into consideration different regions/farming systems, the development of species-specific Codes of Good Aquaculture Practices (CAqP), the importance of staff training by continuous professional development courses (CPDs) and the improvement of the monitoring systems both for the aquatic environment and the behaviour, health and welfare of the farmed animals. A systematic integrated welfare assessment encompassing all the different aspects of their farming and life cycle up to slaughter should be implemented in all aquaculture systems to better understand the basic needs of these organisms, to reduce the stressors, which would minimise the effects of stress responses and improve productivity and quality of the final product. The development of the precision farming (PF), with real-time monitoring of the environmental parameters and the possibility of system modelling and machine learning is a step forward for aquaculture systems and the solution for many welfare/stress related issues. Direct and indirect species-specific OWIs should be developed for all decapod crustaceans currently farmed, similar to the ones suggested in this review for P. vannamei. Positive welfare using environmental enrichment and better and less invasive husbandry procedures needs to be promoted at all life stages and husbandry practices, from broodstock to slaughter methods.
Understanding sentience in invertebrates is important from an animal and scientific perspective (Rowe, 2018). Moreover, as pointed out by Birch (Birch et al., 2021) evidence of sentience in invertebrates is crucial from a policy perspective. For instance, in the European Union decapod crustaceans were not included in the EU Directive 2010/63/EU on the protection of animals used for scientific purposes, nor other national directives such as the UK’s Animal Welfare Act 2006. However, protection of decapod crustaceans has been argued for a number of years and therefore it is possible that the scope of current regulations will be expanded to decapod species. This has already happened in a number of cases such as in the New Zealand’s Animal Welfare Act (1999), which includes crabs, lobsters and crayfish, while in Norway legislation includes all decapods as well as some insects (honeybees). Regarding the use of decapod species for scientific purposes, again while there are country- and even institution-specific differences, the requirement for ethical review has been firmly proposed (Rowe, 2018). More research related to crustacean welfare therefore needs to be developed to inform the industry. The new focus on welfare in crustaceans should be seen as an opportunity to boost the farming industry and address the needs of consumers.
Author Contributions
All authors listed have made a substantial, direct, and intellectual contribution to the work, and approved it for publication.
Conflict of Interest
The authors declare that the research was conducted in the absence of any commercial or financial relationships that could be construed as a potential conflict of interest.
Publisher’s Note
All claims expressed in this article are solely those of the authors and do not necessarily represent those of their affiliated organizations, or those of the publisher, the editors and the reviewers. Any product that may be evaluated in this article, or claim that may be made by its manufacturer, is not guaranteed or endorsed by the publisher.
Acknowledgments
CC is joint-lead for WP3 Shellfish Health and Disease as part of the BBSRC/NERC-funded Aquaculture Collaboration Hub UK.
Footnotes
- ^ World Organisation for Animal Health (OiE) 2022. Aquatics, Crustaceans. Available at: https://www.oie.int/en/what-we-do/animal-health-and-welfare/animal-diseases/?_tax_animal=aquatics%2Ccrustaceans
- ^ https://www.globalseafood.org/advocate/investment-firm-paper-based-disease-test-kits/
- ^ https://www.innovationnewsnetwork.com/ensuring-fish-welfare-in-aquaculture-with-new-digital-app/12855/
- ^ https://www.asc-aqua.org/wp-content/uploads/2019/03/ASC-Shrimp-Standard_v1.1_Final.pdf
- ^ ASIC: http://www.asicollaborative.org/
- ^ BAP: https://bapcertification.org/blog/category/shrimp/
- ^ https://asean.org/wp-content/uploads/images/archive/AMAF%2033%20Standard%20on%20ASEAN%20Shrimp%20GAP.pdf
- ^ API: http://api.worldanimalprotection.org/sites/default/files/api_2020_-_australia.pdf
- ^ The Federal Act on Animal Welfare (Tierschutzgesetz - TSchG), Federal Law Gazette I 2004/118
- ^ https://api.worldanimalprotection.org/sites/default/files/api_2020_-_austria_0.pdf
- ^ https://www.globalanimallaw.org/downloads/database/national/switzerland/TSchV-2008-EN-455.1-2011.pdf
- ^ https://www.legislation.govt.nz/act/public/1999/0142/latest/DLM49664.html
- ^ https://www.regjeringen.no/en/dokumenter/animal-welfare-act/id571188/#:~:text=The%20intention%20of%20this%20Act,welfare%20and%20respect%20for%20animals.&text=The%20Act%20applies%20to%20conditions,squid%2C%20octopi%20and%20honey%20bees
References
Albalat A., Gornik S. G., Atkinson R. J., Coombs G. H., Neil D. M. (2009). Effect of Capture Method on the Physiology and Nucleotide Breakdown Products in the Norway Lobster (Nephrops Norvegicus). Mar. Biol. Res. 5, 441–450. doi: 10.1080/17451000802603637
Albalat A., Gornik S., Muangnapoh C., Neil D.M. (2022). Effectiveness and quality evaluation of electrical stunning versus chilling in Norway lobsters (Nephrops norvegicus). Food Control 138 (9), 108930. doi: /10.1016/j.foodcont.2022.108930
Albalat A., Johnson L., Coates C. J., Dykes G. C., Hitte F., Morro B., et al. (2019). The Effect of Temperature on the Physiological Condition and Immune-Capacity of European Lobsters (Homarus Gammarus) During Long-Term Starvation. Front. Mar. Sci. 6, 281. doi: 10.3389/fmars.2019.00281
Albalat A., Sinclair S., Neil D. (2017). Validation of a Vigour Index for Trawl-Caught Norway Lobsters (Nephrops Norvegicus) Destined for the Live Market: Underlying Links to Both Physiological Condition and Survivability. Fish. Res. 191, 25–29. doi: 10.1016/j.fishres.2017.02.016
Animal Health and Animal Welfare Panel of the European Food Standards Agency [AHAW]. (2005). Aspects of the Biology and Welfare of Animals Used for Experimental and Other Scientific Purposes (EFSA-Q-2004-105). Annex EFSA J. 292, 1–136. doi: 10.2903/j.efsa.2005.292
Araneda M., Gasca-Leyva E., Vela M. A., Dominguez-May R. (2020). Effects of Temperature and Stocking Density on Intensive Culture of Pacific White Shrimp in Freshwater. J. Therm. Biol. 94, 102756. doi: 10.1016/j.jtherbio.2020.102756
Arechavala-Lopez P., Cabrera-Álvarez M. J., Maia C. M., Saraiva J. L. (2022). Environmental Enrichment in Fish Aquaculture: A Review of Fundamental and Practical Aspects. Rev. Aquac. 14, 704–728. doi: 10.1111/raq.12620
Arnold J. E., Hadfield C. A., Clayton L. A., Cray C., Jones D., Payton M. (2021). Development of Methodology and Reference Intervals for the Analysis of the Free-Ranging Atlantic Horseshoe Crab Limulus Polyphemus Hemolymph. Vet. Clin. Pathol. 50, 259–272. doi: 10.1111/vcp.12983
Bachère E. (2000). Shrimp Immunity and Disease Control. Aquaculture 191, 3–11. doi: 10.1016/S0044-8486(00)00413-0
Bae S.-H., Okutsu T., Kang B. J., Wilder M. N. (2013). Alterations of Pattern in Immune Response and Vitellogenesis During Induced Ovarian Development by Unilateral and Bilateral Ablation in Litopenaeus Vannamei. Fish. Sci. 79, 895–903. doi: 10.1007/s12562-013-0652-3
Bardera G., Owen M. A. G., Facanha F. N., Alcaraz-Calero J. M., Alexander M. E., Sloman K. A. (2021). The Influence of Density and Dominance on Pacific White Shrimp (Litopenaeus Vannamei) Feeding Behaviour. Aquaculture 531, 735949. doi: 10.1016/j.aquaculture.2020.735949
Bardera G., Owen M. A. G., Façanha F. N., Sloman K. A., Alexander M. E. (2020). The Influence of Sex on Feeding Behaviour in Pacific White Shrimp (Litopenaeus Vannamei). Appl. Anim. Behav. Sci. 224, 104946. doi: 10.1016/j.applanim.2020.104946
Bardera G., Usman N., Owen M., Pountney D., Sloman K. A., Alexander M. E. (2019). The Importance of Behaviour in Improving the Production of Shrimp in Aquaculture. Rev. Aquac. 11, 1104–1132. doi: 10.1111/raq.12282
Barreto M. O., Rey Planellas S., Yang Y., Phillips C., Descovich K. (2022). Emerging Indicators of Fish Welfare in Aquaculture. Rev. Aquac. 14, 343–361. doi: 10.1111/raq.12601
Bartlett T. C., Cuthbertson B. J., Shepard E. F., Chapman R. W., Gross P. S., Warr G. W. (2002). Crustins, Homologues of an 11.5-Kda Antibacterial Peptide, From Two Species of Penaeid Shrimp, Litopenaeus Vannamei and Litopenaeus Setiferus. Mar. Biotechnol. 4, 278–293. doi: 10.1007/s10126-002-0020-2
Basti D., Bricknell I., Chang E. S., Bouchard D. (2010). Biochemical Reference Intervals for the Resting State in the Adult Lobster Homarus Americanus. J. Shellfish Res. 29, 1013–1019, 1017.
Bateson P. (1991). Assessment of Pain in Animals. Anim. Behav. 42, 827–839. doi: 10.1016/S0003-3472(05)80127-7
Bautista-Covarrubias J. C., Zamora-Ibarra P. A., Apreza-Burgos E., Rodríguez-Ocampo A. N., Peraza-Gómez V., López-Sánchez J. A., et al. (2020). Immune Response and Oxidative Stress of Shrimp Litopenaeus Vannamei at Different Moon Phases. Fish. Shellfish Immunol. 106, 591–595. doi: 10.1016/j.fsi.2020.08.040
Becker A. J., Vaz L. J., Garcia L., Wasielesky J., Heinzmann B. M., Baldisserotto B. (2021). Anesthetic Potential of Different Essential Oils for Two Shrimp Species, Farfantepenaeus Paulensis and Litopenaeus Vannamei (Decapoda, Crustacea). Ciec. Rural 51, 12, e20200793. doi: 10.1590/0103-8478cr20200793
Birch J. B., Schnell C., Browning A., H. Crump A. (2021). Review of the Evidence of Sentience in Cephalopod Molluscs and Decapod Crustaceans, London School of Economics and Political Science (London, UK: LSE Enterprise Ltd; London School of Economics and Political Science).
Bonami J.-R., Hasson K. W., Mari J., Poulos B. T., Lightner D. V. (1997). Taura Syndrome of Marine Penaeid Shrimp: Characterization of the Viral Agent. J. Gen. Virol. 78, 313–319. doi: 10.1099/0022-1317-78-2-313
Bonami J. R., Shi Z., Qian D., Sri Widada J. (2005). White Tail Disease of the Giant Freshwater Prawn, Macrobrachium Rosenbergii: Separation of the Associated Virions and Characterization of MRNV as a New Type of Nodavirus. J. Fish. Dis. 28, 23–31. doi: 10.1111/j.1365-2761.2004.00595.x
Borquez-Lopez R. A., Martinez-Cordova L. R., Casillas-Hernandez R., Lopez-Elias J. A., Barraza-Guardado R. H., Ibarra-Gamez J. C., et al. (2016). Water Quality Index Monitoring for Shrimp Aquaculture Using Open Source Hardware and Fuzzy Inference Systems. Biotecnia 19, 3, 45–49. doi: 10.18633/biotecnia.v19i3.449
Broom D. M. (2019). “Sentience,” in Encyclopedia of Animal Behavior, 2nd ed. Ed. Choe J. C. (Oxford: Academic Press), 131–133, pp.
Browdy C. L. (1998). Recent Developments in Penaeid Broodstock and Seed Production Technologies: Improving the Outlook for Superior Captive Stocks. Aquaculture 164, 3–21. doi: 10.1016/S0044-8486(98)00174-4
Burgents J. E., Burnett K. G., Burnett L. E. (2005). Effects of Hypoxia and Hypercapnic Hypoxia on the Localization and the Elimination of Vibrio Campbellii in Litopenaeus Vannamei, the Pacific White Shrimp. Biol. Bull. 208, 159–168. doi: 10.2307/3593148
Butterworth A., Weeks C. (2009). The Impact of Disease on Welfare. Welf. Domest. Fowl Other Captive Birds 9, 189–218. doi: 10.1007/978-90-481-3650-6_8
Calderon-Perez J. A., Rendon-Rodriguez S., Solis-Ibarra R. (2007). Daily Cycle and Body Characteristics of Mating Litopenaeus Vannamei Shrimps (Decapoda: Penaeidae) in the Wild Off Southern Sinaloa, Mexico. Rev. Biol. Trop. 55, 189–198. doi: 10.15517/rbt.v55i1.6070
Cerenius L., Babu R., Söderhäll K., Jiravanichpaisal P. (2010). In Vitro Effects on Bacterial Growth of Phenoloxidase Reaction Products. J. Invertebr. Pathol. 103, 21–23. doi: 10.1016/j.jip.2009.09.006
Cerenius L., Söderhäll K. (2021). Immune Properties of Invertebrate Phenoloxidases. Dev. Comp. Immunol. 122, 104098. doi: 10.1016/j.dci.2021.104098
Chaijarasphong T., Munkongwongsiri N., Stentiford G. D., Aldama-Cano D. J., Thansa K., Flegel T. W., et al. (2021). The Shrimp Microsporidian Enterocytozoon Hepatopenaei (EHP): Biology, Pathology, Diagnostics and Control. J. Invertebr. Pathol. 186, 107458. doi: 10.1016/j.jip.2020.107458
Chamberlain G. W., Lawrence A. L. (1981). Maturation, Reproduction, and Growth of Penaeus Vannamei and P Stylirostris Fed Natural Diets. J. World Mariculture Soc. 12, 207–224. doi: 10.1111/j.1749-7345.1981.tb00256.x
Cheng W., Wang L.-U., Chen J.-C. (2005). Effect of Water Temperature on the Immune Response of White Shrimp Litopenaeus Vannamei to Vibrio Alginolyticus. Aquaculture 250, 592–601. doi: 10.1016/j.aquaculture.2005.04.060
Ciaramella M. A., Battison A. L., Horney B. (2014). Measurement of Tissue Lipid Reserves in the American Lobster (Homarus Americanus): Hemolymph Metabolites as Potential Biomarkers of Nutritional Status. J. Crust. Biol. 34, 629–638. doi: 10.1163/1937240X-00002262
Coates C. J., Bradford E. L., Krome C. A., Nairn J. (2012). Effect of Temperature on Biochemical and Cellular Properties of Captive Limulus Polyphemus. Aquaculture 334-337, 30–38. doi: 10.1016/j.aquaculture.2011.12.029
Coates C. J., Costa-Paiva E. M. (2020). “Multifunctional Roles of Hemocyanins,” in Vertebrate and Invertebrate Respiratory Proteins, Lipoproteins and Other Body Fluid Proteins. Eds. Hoeger U., Harris J. R. (Cham: Springer International Publishing), pp 233–pp 250.
Coates C. J., Nairn J. (2013). Hemocyanin-Derived Phenoloxidase Activity: A Contributing Factor to Hyperpigmentation in Nephrops Norvegicus. Food Chem. 140, 361–369. doi: 10.1016/j.foodchem.2013.02.072
Coates C. J. R., Rowley A. F., Smith L. C., Whitten M. M. A. (2022). “Host Defences of Invertebrates to Pathogens and Parasites,” in Invertebrate Pathology. Eds. Rowley A. F., Coates C. J., Miranda M. A. (Oxford UK: Oxford University Press).
Coates C. J., Söderhäll K. (2021). The Stress–Immunity Axis in Shellfish. J. Invertebr. Pathol. 186, 107492. doi: 10.1016/j.jip.2020.107492
Coates C. J., Talbot J. (2018). Hemocyanin-Derived Phenoloxidase Reaction Products Display Anti-Infective Properties. Dev. Comp. Immunol. 86, 47–51. doi: 10.1016/j.dci.2018.04.017
Conte F., Voslarova E., Vecerek V., Elwood R. W., Coluccio P., Pugliese M., et al. (2021). Humane Slaughter of Edible Decapod Crustaceans. Animals 11, 1089. doi: 10.3390/ani11041089
Cook H. L., Murphy M. A. (1969). The Culture of Larval Penaeid Shrimp. Trans. Am. Fish. Soc. 98, 751–754. doi: 10.1577/1548-8659(1969)98[751:TCOLPS]2.0.CO;2
Costa A. M., Buglione C. C., Bezerra F. L., Martins P. C. C., Barracco M. A. (2009). Immune Assessment of Farm-Reared Penaeus Vannamei Shrimp Naturally Infected by Imnv in Ne Brazil. Aquaculture 291, 141–146. doi: 10.1016/j.aquaculture.2009.03.013
Cummings W. C. (1961). Maturation and Spawning of the Pink Shrimp, Penaeus Duorarum Burkenroad. Trans. Am. Fish. Soc. 90, 462–468. doi: 10.1577/1548-8659(1961)90[462:MASOTP]2.0.CO;2
Cuvin-Aralar M. L. A., Lazartigue A. G., Aralar E. V. (2009). Cage Culture of the Pacific White Shrimp Litopenaeus Vannamei (Boon) at Different Stocking Densities in a Shallow Eutrophic Lake. Aquac. Res. 40, 181–187. doi: 10.1111/j.1365-2109.2008.02081.x
da Costa F. P., Gomes B., Pereira S., Arruda M. D. (2016). Influence of Stocking Density on the Behaviour of Juvenile Litopenaeus Vannamei (Boon). Aquac. Res. 47, 912–924. doi: 10.1111/are.12550
D’Agaro E., Sabbioni V., Messina M., Tibaldi E., Bongiorno T., Tulli F., et al. (2014). Effect of Confinement and Starvation on Stress Parameters in the American Lobster (Homarus Americanus). Ital. J. Anim. Sci. 13, 3530. doi: 10.4081/ijas.2014.3530
Darwin C. (1872). The Expression of the Emotions in Man and Animals. Ed. Murray J. (London, UK: John Murray).
Das R., Krishna G., Priyadarshi H., P G.-B., Pavan-Kumar A., Rajendran K. V., et al. (2015). Captive Maturation Studies in Penaeus Monodon by Gih Silencing Using Constitutively Expressed Long Hairpin Rna. Aquaculture 448, 512–520. doi: 10.1016/j.aquaculture.2015.06.036
Davies C. E., Malkin S. H., Thomas J. E., Batista F. M., Rowley A. F., Coates C. J. (2020). Mycosis is a Disease State Encountered Rarely in Shore Crabs, Carcinus Maenas. Pathog. (Basel Switzerland) 9, 462. doi: 10.3390/pathogens9060462
De Gryse G. M. A., Khuong T. V., Descamps B., Van Den Broeck W., Vanhove C., Cornillie P., et al. (2020). The Shrimp Nephrocomplex Serves as a Major Portal of Pathogen Entry and is Involved in the Molting Process. Proc. Natl. Acad. Sci. 117, 28374–28383. doi: 10.1073/pnas.2013518117
de Souza Valente C., Wan A. H. L. (2021). Vibrio and Major Commercially Important Vibriosis Diseases in Decapod Crustaceans. J. Invertebr. Pathol. 181, 107527. doi: 10.1016/j.jip.2020.107527
Diamond S., Powell A., Shields R. J., Rowley A. F. (2008). Is Spermatophore Melanisation in Captive Shrimp (Litopenaeus Vannamei) a Result of an Auto-Immune Response? Aquaculture 285, 14–18. doi: 10.1016/j.aquaculture.2008.08.029
Diggles B. K. (2019). Review of Some Scientific Issues Related to Crustacean Welfare. ICES J. Mar. Sci. 76, 66–81. doi: 10.1093/icesjms/fsy058
Durairaj K., Velmurugan P., Senthilkumar P., Vetriselvan P., Manimekalan A., Oh B. T. (2018). In-Vivo Study on Effect of Stocking Density on Growth and Production of Marine Prawn Litopenaeus Vannamei. Indian J. Geo-Mar. Sci. 47, 1228–1236.
Elwald J. J. (1965). The Laboratory Rearing of Pink Shrimp, Penaeus Duorarum Burkenroad. Bull. Mar. Sci. Gulf Caribb. 15, 436–449.
Esparza-Leal H. M., Cardozo A. P., Wasielesky W. (2015). Performance of Litopenaeus Vannamei Postlarvae Reared in Indoor Nursery Tanks at High Stocking Density in Clear-Water Versus Biofloc System. Aquacult. Eng. 68, 28–34. doi: 10.1016/j.aquaeng.2015.07.004
FAO. (2003). Health Management and Biosecurity Maintenance in White Shrimp (Penaeus Vannamei) Hatcheries in Latin America (Rome, Italy: Food and Agriculture Organization of the United Nations).
FAO. (2009). “Penaeus Vannamei,” in Cultured Aquatic Species Fact Sheets. Eds. Briggs M., Valerio C., Michael N. (Rome, Italy: Food and Agriculture Organisation of the United Nations).
FAO. (2020). The State of World Fisheries and Aquaculture 2020, Sustainability in Action (Rome: FAO).
Fontaine C. T., Lightner D. V. (1973). Observations on the Process of Wound Repair in Penaeid Shrimp. J. Invertebr. Pathol. 22, 23–33. doi: 10.1016/0022-2011(73)90005-0
Fregin T., Bickmeyer U. (2016). Electrophysiological Investigation of Different Methods of Anesthesia in Lobster and Crayfish. PloS One 11, e0162894. doi: 10.1371/journal.pone.0162894
Freire C. A., Cuenca A. L. R., Leite R. D., Prado A. C., Rios L. P., Stakowian N., et al. (2020). Biomarkers of Homeostasis, Allostasis, and Allostatic Overload in Decapod Crustaceans of Distinct Habitats and Osmoregulatory Strategies: An Empirical Approach. Comp. Biochem. Physiol. Part A: Mol. Integr. Physiol. 248, 110750. doi: 10.1016/j.cbpa.2020.110750
Frelier P. F., Loy J. K., Kruppenbach B. (1993). Transmission of Necrotizing Hepatopancreatitis in Penaeus Vannamei. J. Invertebr. Pathol. 61, 44–48. doi: 10.1006/jipa.1993.1008
Froes C., Foes G., Krummenauer D., Poersch L. H., Wasielesky W. (2013). Stocking Density at Rearing Phase for White Shrimp Farmed in a Biofloc System. Pesqui. Agropecu. Bras. 48, 878–884. doi: 10.1590/S0100-204X2013000800010
Funge-Smith S., Briggs M. (2003). “The Introduction of Penaeus Vannamei and P Stylirostris Into the Asia-Pacific Region,” in International Mechanisms for the Control and Responsible Use of Alien Species in Aquatic Ecosystems (Rome, Italy: Food and Agriculture Organisation of the United Nations (FAO)) 26–29.
Geetha R., Avunje S., Solanki H. G., Priyadharshini R., Vinoth S., Anand P. R., et al. (2022). Farm-Level Economic Cost of Enterocytozoon Hepatopenaei (EHP) to Indian Penaeus Vannamei Shrimp Farming. Aquaculture 548, 737685. doi: 10.1016/j.aquaculture.2021.737685
Gong Y., Zhang X. (2021). Rnai-Based Antiviral Immunity of Shrimp. Dev. Comp. Immunol. 115, 103907. doi: 10.1016/j.dci.2020.103907
Gornik S. G., Albalat A., Atkinson R. J., Coombs G. H., Neil D. M. (2010). The Influence of Defined Ante-Mortem Stressors on the Early Post-Mortem Biochemical Processes in the Abdominal Muscle of the Norway Lobster, Nephrops Norvegicus (Linnaeu). Mar. Biol. Res. 6, 223–238. doi: 10.1080/17451000903147468
Gunalan B., Soundarapandian P., Dinakaran G. K. (2010). The Effect of Temperature and Ph on WSSV Infection in Cultured Marine Shrimp Penaeus Monodon (Fabricius). Middle-East J. Sci. Res. 5, 28–33.
Hasson K. W., Lightner D. V., Poulos B. T., Redman R. M., White B. L., Brock J. A., et al. (1995). Taura Syndrome in Penaeus Vannamei: Demonstration of a Viral Etiology. Dis. Aquat. Org. 23, 115–126. doi: 10.3354/dao023115
Hauton C., Hawkins L., Williams J. (1997). In Situ Variability in Phenoloxidase Activity in the Shore Crab, Carcinus Maenas (L.). Comp. Biochem. Physiol. Part B: Biochem. Mol. Biol. 117, 267–271. doi: 10.1016/S0305-0491(97)00050-3
Heng L., Rui-yu L. (1994). Comparative Studies on the Larval Development of the Penaeid Shrimps, Penaeus Chinensis, P Merguiensis and P Penicillatus. Chin. J. Oceanol. Limnol. 12, 295–307. doi: 10.1007/BF02850489
Holt C. C., Bass D., Stentiford G. D., van der Giezen M. (2021). Understanding the Role of the Shrimp Gut Microbiome in Health and Disease. J. Invertebr. Pathol. 186, 107387. doi: 10.1016/j.jip.2020.107387
Huang S., Kuang Y., Chang C., Hung C., Tsai C., Feng K. (2019). AIo Ts for Smart Shrimp Farming International SoC Design Conference, Jeju, South Korea: ISOCC, 17–18, pp.
Hudinaga M. (1935). Studies on the Development of Penaeus Japonicus Bate: 1st Report (Hayatomo, Japan: Hayatomo Fishery Institute).
Hudinaga M. G. K. K. (1942). Reproduction, Development and Rearing of Penaeus Japonicus Bate (Tokyo: National Research Council of Japan).
Hudinaga M. K. J. (1966). Studies on Food and Growth of Larval Stage of a Prawn, Penaeus Japonicus, With Reference to the Application to Practical Mass Culture. Inf. Bull. Planktol. Jpn. 13, 83–94.
Hudinaga M., Kittaka J. (1967). The Large Scale Production of the Young Kuruma Prawn, Penaeus Japonicus Bate. Inf. Bull. Planktol. Jpn. (Commemoration No. Dr. Y. Matsue's 60th Birthday), 35–46.
Hu J., Wang S., Wang L., Li F., Pingguan-Murphy B., Lu T. J., et al. (2014). Advances in Paper-Based Point-of-Care Diagnostics. Biosens. Bioelectron. 54, 585–597. doi: 10.1016/j.bios.2013.10.075
Jerez-Cepa I., Ruiz-Jarabo I. (2021). Physiology: An Important Tool to Assess the Welfare of Aquatic Animals. Biology 10, 61. doi: 10.3390/biology10010061
Johansson M. W., Keyser P., Sritunyalucksana K., Söderhäll K. (2000). Crustacean Haemocytes and Haematopoiesis. Aquaculture 191, 45–52. doi: 10.1016/S0044-8486(00)00418-X
Johnson L., Coates C. J., Albalat A., Todd K., Neil D. (2016). Temperature-Dependent Morbidity of ‘Nicked’ Edible Crab, Cancer Pagurus. Fish. Res. 175, 127–131. doi: 10.1016/j.fishres.2015.11.024
Jorry D. C. (2012). “Marine Shrimps,” in Aquaculture: Farming Aquatic Animals and Plants. Eds. Lucas J. S., Southgate P. C. (Australia: John Wiley & sons), 382–418, pp.
Kang B. J., Okutsu T., Tsutsui N., Shinji J., Bae S.-H., Wilder M. N. (2014). Dynamics of Vitellogenin and Vitellogenesis-Inhibiting Hormone Levels in Adult and Subadult Whiteleg Shrimp, Litopenaeus Vannamei: Relation to Molting and Eyestalk Ablation1. Biol. Reprod. 90, 1, 1–10. doi: 10.1095/biolreprod.113.112243
Kirkwood J. K. (2006). “The Distribution of the Capacity for Sentience in the Animal Kingdom,” in Animals, Ethics and Trade: The Challenge of Animal Sentience. Eds. Turner J., D'Silva J. (Petersfield: Compassion in World Farming Trust), 12–26, pp.
Krisfalusi-Gannon J., Ali W., Dellinger K., Robertson L., Brady T. E., Goddard M. K. M., et al. (2018). The Role of Horseshoe Crabs in the Biomedical Industry and Recent Trends Impacting Species Sustainability. Front. Mar. Sci. 5, 185. doi: 10.3389/fmars.2018.00185
Kumar B. K., Deekshit V. K., Raj J. R. M., Rai P., Shivanagowda B. M., Karunasagar I., et al. (2014). Diversity of Vibrio Parahaemolyticus Associated With Disease Outbreak Among Cultured Litopenaeus Vannamei (Pacific White Shrimp) in India. Aquaculture 433, 247–251. doi: 10.1016/j.aquaculture.2014.06.016
Kumar V., Roy S., Baruah K., Van Haver D., Impens F., Bossier P. (2020). Environmental Conditions Steer Phenotypic Switching in Acute Hepatopancreatic Necrosis Disease-Causing Vibrio Parahaemolyticus, Affecting Piravp/Pirbvp Toxins Production. Environ. Microbiol. 22, 4212–4230. doi: 10.1111/1462-2920.14903
Liao X., Wang C., Wang B., Qin H., Hu S., Wang P., et al. (2020). Comparative Transcriptome Analysis of Litopenaeus Vannamei Reveals That Triosephosphate Isomerase-Like Genes Play an Important Role During Decapod Iridescent Virus 1 Infection. Front. Immunol. 11, 1904. doi: 10.3389/fimmu.2020.01904
Li E., Chen L., Zeng C., Yu N., Xiong Z., Chen X., et al. (2008). Comparison of Digestive and Antioxidant Enzymes Activities, Haemolymph Oxyhemocyanin Contents and Hepatopancreas Histology of White Shrimp, Litopenaeus Vannamei, at Various Salinities. Aquaculture 274, 80–86. doi: 10.1016/j.aquaculture.2007.11.001
Lightner D. V., Pantoja C. R., Poulos B. T., Tang K. F. J., Redman R. M., Andrade T. P. D., et al. (2004). Infectious Myonecrosis: New Disease in Pacific White Shrimp. Glob Aquac Advocate 7, 85.
Lightner D. V., Redman R. M., Bell T. A. (1983). Infectious Hypodermal and Hematopoietic Necrosis, a Newly Recognized Virus Disease of Penaeid Shrimp. J. Invertebr. Pathol. 42, 62–70. doi: 10.1016/0022-2011(83)90202-1
Lightner D. V., Redman R. M., Pantoja C. R., Noble B. L., Loc T. (2012). Early Mortality Syndrome Affects Shrimp in Asia. (Portsmouth, U.S.A.: Global Seafood Alliance) 40.
Linda N., Wiwat S.-o., Supapon C., Timothy W. F. (2005). Taura Syndrome Virus (TSV) in Thailand and its Relationship to TSV in China and the Americas. Dis. Aquat. Org. 63, 101–106. doi: 10.3354/dao063101
Liu F., Li S., Yu Y., Zhang C., Li F. (2021). Antennal Gland of Shrimp as an Entry for WSSW Infection. Aquaculture 530, 735932. doi: 10.1016/j.aquaculture.2020.735932
Liu C.-H., Yeh S.-T., Cheng S.-Y., Chen J.-C. (2004). The Immune Response of the White Shrimp Litopenaeus Vannamei and its Susceptibility to Vibrio Infection in Relation With the Moult Cycle. Fish. Shellfish Immunol. 16, 151–161. doi: 10.1016/S1050-4648(03)00058-5
Liu S., Zheng S.-C., Li Y.-L., Li J., Liu H.-P. (2020). Hemocyte-Mediated Phagocytosis in Crustaceans. Front. Immunol. 11, 268. doi: 10.3389/fimmu.2020.00268
Li E., Xu C., Wang X., Wang S., Zhao Q., Zhang M., et al. (2018). Gut Microbiota and its Modulation for Healthy Farming of Pacific White Shrimp Litopenaeus Vannamei. Rev. Fish. Sci. Aquac. 26, 381–399. doi: 10.1080/23308249.2018.1440530
Magaña-Gallegos E., Arévalo M., Cuzon G., Gaxiola G. (2021). Effects of Using the Biofloc System and Eyestalk Ablation on Reproductive Performance and Egg Quality of Litopenaeus Vannamei (Boon) (Decapoda: Dendrobranchiata: Penaeidae). Anim. Reprod. Sci. 228, 106749. doi: 10.1016/j.anireprosci.2021.106749
Maningas M. B. B., Kondo H., Hirono I. (2013). Molecular Mechanisms of the Shrimp Clotting System. Fish. Shellfish Immunol. 34, 968–972. doi: 10.1016/j.fsi.2012.09.018
Martins C. I. M., Galhardo L., Noble C., Damsgård B., Spedicato M. T., Zupa W., et al. (2012). Behavioural Indicators of Welfare in Farmed Fish. Fish. Physiol. Biochem. 38, 17–41. doi: 10.1007/s10695-011-9518-8
Mellor D. J., Beausoleil N. J. (2015). Extending the ‘Five Domains’ Model for Animal Welfare Assessment to Incorporate Positive Welfare States. Anim. Welf. 24, 241. doi: 10.7120/09627286.24.3.241
Mellor D. J., Patterson-Kane E., Stafford K. J. (2009). The Sciences of Animal Welfare (Oxford, UK: Wiley-Blackwell).
Mellor D., Reid C. (1994). Concepts of Animal Well-Being and Predicting the Impact of Procedures on Experimental Animals. Improv. Well-Being Anim. Res. Environ., 3–18. https://www.wellbeingintlstudiesrepository.org/exprawel/7/
Metian M., Troell M., Christensen V., Steenbeek J., Pouil S. (2020). Mapping Diversity of Species in Global Aquaculture. Rev. Aquac. 12, 1090–1100. doi: 10.1111/raq.12374
Millard R. S., Ellis R. P., Bateman K. S., Bickley L. K., Tyler C. R., van Aerle R., et al. (2021). How Do Abiotic Environmental Conditions Influence Shrimp Susceptibility to Disease? A Critical Analysis Focussed on White Spot Disease. J. Invertebr. Pathol. 186, 107369. doi: 10.1016/j.jip.2020.107369
Mohr P. G., Moody N. J., Hoad J., Williams L. M., Bowater R. O., Cummins D. M., et al. (2015). New Yellow Head Virus Genotype (Yhv7) in Giant Tiger Shrimp Penaeus Monodon Indigenous to Northern Australia. Dis. Aquat. Org. 115, 263–268. doi: 10.3354/dao02894
Muthu M. S., Pillai N. N., George K. V. (1974). On the Spawning and Rearing of Penaeus Indicus in the Laboratory With a Note on the Eggs and Larvae. Indian J. Fish. 21, 571–574.
Neil D. M. (2010). The Effect of the Crustastun™ on Nerve Activity in Crabs and Lobsters (Glasgow, UK: University of Glasgow).
Neil D. M. (2012a). “The Effect of the Crustastun™ on Nerve Activity in Two Commercially Important Decapod Crustaceans,” in The Edible Brown Cancer Pagurus and the European Lobster Homarus Gammarus (Glasgow, UK: University of Glasgow).
Neil D. M. (2012b). Ensuring Crustacean Product Quality in the Post-Harvest Phase. J. Invertebr. Pathol. 110, 267–275. doi: 10.1016/j.jip.2012.03.009
Ng T. H., Chang S.-H., Wu M.-H., Wang H.-C. (2013). Shrimp Hemocytes Release Extracellular Traps That Kill Bacteria. Dev. Comp. Immunol. 41, 644–651. doi: 10.1016/j.dci.2013.06.014
Noble C., Gismervik K., Iversen M. H., Kolarevic J., Nilsson J., Stien L. H., et al. (2018). Welfare Indicators for Farmed Atlantic Salmon: Tools for Assessing Fish Welfare (Tromsø, Norway: Nofima).
Nunan L. M., Pantoja C. R., Gomez-Jimenez S., Lightner D. V. (2013). Candidatus Hepatobacter Penaei: An Intracellular Pathogenic Enteric Bacterium in the Hepatopancreas of the Marine Shrimp Penaeus Vannamei (Crustacea: Decapoda). Appl. Environ. Microbiol. 79, 1407–1409. doi: 10.1128/AEM.02425-12
O'Donncha F., Stockwell C. L., Planellas S. R., Micallef G., Palmes P., Webb C., et al. (2021). Data Driven Insight Into Fish Behaviour and Their Use for Precision Aquaculture. Front. Anim. Sci. 30, 695054. doi: 10.3389/fanim.2021.695054
Palacios E., Carrefio D., Rodríguez-Jaramillo M. C., Racotta I. S. (1999a). Effect of Eyestalk Ablation on Maturation, Larval Performance, and Biochemistry of White Pacific Shrimp, Penaeus Vannamei, Broodstock. J. Appl. Aquac. 9, 1–23. doi: 10.1300/J028v09n03_01
Palacios E., Perez-Rostro C. I., Ramirez J. L., Ibarra A. M., Racotta I. S. (1999b). Reproductive Exhaustion in Shrimp (Penaeus Vannamei) Reflected in Larval Biochemical Composition, Survival and Growth. Aquaculture 171, 309–321. doi: 10.1016/S0044-8486(98)00393-7
Parodi T. V., Cunha M. A., Heldwein C. G., de Souza D. M., Martins Á. C., Garcia L., et al. (2012). The Anesthetic Efficacy of Eugenol and the Essential Oils of Lippia Alba and Aloysia Triphylla in Post-Larvae and Sub-Adults of Litopenaeus Vannamei (Crustacea, Penaeidae). Comp. Biochem. Physiol. Part C: Toxicol. Pharmacol. 155, 462–468. doi: 10.1016/j.cbpc.2011.12.003
Passantino A., Elwood R. W., Coluccio P. (2021). Why Protect Decapod Crustaceans Used as Models in Biomedical Research and in Ecotoxicology? Ethical and Legislative Considerations. Animals 11, 73. doi: 10.3390/ani11010073
Phupet B., Pitakpornpreecha T., Baowubon N., Runsaeng P., Utarabhand P. (2018). Lipopolysaccharide- and β-1,3-Glucan-Binding Protein From Litopenaeus Vannamei: Purification, Cloning and Contribution in Shrimp Defense Immunity via Phenoloxidase Activation. Dev. Comp. Immunol. 81, 167–179. doi: 10.1016/j.dci.2017.11.016
Poulos B. T., Tang K. F. J., Pantoja C. R., Bonami J. R., Lightner D. V. (2006). Purification and Characterization of Infectious Myonecrosis Virus of Penaeid Shrimp. J. Gen. Virol. 87, 987–996. doi: 10.1099/vir.0.81127-0
Prachumwat A., Munkongwongsiri N., Eamsaard W., Lertsiri K., Flegel T. W., Stentiford G. D., et al. (2021). A Potential Prokaryotic and Microsporidian Pathobiome That may Cause Shrimp White Feces Syndrome (WFS). bioRxiv 2005, 2023.445355. doi: 10.1101/2021.05.23.445355
Prasad K. P., Shyam K. U., Banu H., Jeena K., Krishnan R. (2017). Infectious Myonecrosis Virus (IMNV) – an Alarming Viral Pathogen to Penaeid Shrimps. Aquaculture 477, 99–105. doi: 10.1016/j.aquaculture.2016.12.021
Qiu L., Chen X., Guo X.-M., Gao W., Zhao R.-H., Zhang Q.-L., et al. (2020). A Taqman Probe Based Real-Time Pcr for the Detection of Decapod Iridescent Virus 1. J. Invertebr. Pathol. 173, 107367. doi: 10.1016/j.jip.2020.107367
Qi C., Wang L., Liu M., Jiang K., Wang M., Zhao W., et al. (2017). Transcriptomic and Morphological Analyses of Litopenaeus Vannamei Intestinal Barrier in Response to Vibrio Paraheamolyticus Infection Reveals Immune Response Signatures and Structural Disruption. Fish. Shellfish Immunol. 70, 437–450. doi: 10.1016/j.fsi.2017.09.004
Quackenbush L. S. (2015). Yolk Synthesis in the Marine Shrimp, Penaeus Vannamei. Am. Zool. 41, 458–464. doi: 10.1093/icb/41.3.458
Racotta I. S., Palacios E., Ibarra A. M. (2003). Shrimp Larval Quality in Relation to Broodstock Condition. Aquaculture 227, 107–130. doi: 10.1016/S0044-8486(03)00498-8
Rahman M., Escobedo-Bonilla C. M., Corteel M., Dantas-Lima J. J., Wille M., Sanz V. A., et al. (2006). Effect of High Water Temperature (33 °C) on the Clinical and Virological Outcome of Experimental Infections With White Spot Syndrome Virus (WSSV) in Specific Pathogen-Free (SPF) Litopenaeus Vannamei. Aquaculture 261, 842–849. doi: 10.1016/j.aquaculture.2006.09.007
Rateni G., Dario P., Cavallo F. (2017). Smartphone-Based Food Diagnostic Technologies: A Review. Sensors 17, 1453. doi: 10.3390/s17061453
Ravi M., Basha A. N., Sarathi M., Idalia H. R., Widada J. S., Bonami J., et al. (2009). Studies on the Occurrence of White Tail Disease (WTD) Caused by Mrnv and Xsv in Hatchery-Reared Post-Larvae of Penaeus Indicus and P Monodon. Aquaculture 292, 117–120. doi: 10.1016/j.aquaculture.2009.03.051
Rey S., Digka N., MacKenzie S. (2015). Animal Personality Relates to Thermal Preference in Wild-Type Zebrafish, Danio Rerio. Zebrafish 12, 243–249. doi: 10.1089/zeb.2014.1076
Robb C. T., Dyrynda E. A., Gray R. D., Rossi A. G., Smith V. J. (2014). Invertebrate Extracellular Phagocyte Traps Show That Chromatin is an Ancient Defence Weapon. Nat. Commun. 5, 4627. doi: 10.1038/ncomms5627
Rodríguez J., Le Moullac G. (2000). State of the Art of Immunological Tools and Health Control of Penaeid Shrimp. Aquaculture 191, 109–119. doi: 10.1016/S0044-8486(00)00421-X
Rosas C., Cooper E., Pascual C., Brito R., Gelabert R., Moreno T., et al. (2004). Indicators of Physiological and Immunological Status of Litopenaeus Setiferus Wild Populations (Crustacea, Penaeidae). Mar. Biol. 145, 401–413. doi: 10.1007/s00227-004-1321-y
Roth B., Øines S. (2010). Stunning and Killing of Edible Crabs (Cancer Pagurus). Anim. Welf. 19, 287–294.
Roth B., Grimsbø E. (2013). Electrical Stunning of Edible Crabs. Nofima Rapportserie (Tromsø, Norway: Nofima), 8 p.
Roth B., Grimsbø E. (2016). Electrical Stunning of Edible Crabs (Cancer Pagurus): From Single Experiments to Commercial Practice. Anim. Welf. 25, 489–497. doi: 10.7120/09627286.25.4.489
Rowe A. (2018). Should Scientific Research Involving Decapod Crustaceans Require Ethical Review? J. Agric. Environ. Ethics 31, 625–634. doi: 10.1007/s10806-018-9750-7
Sainz-Hernández J. C., Racotta I. S., Dumas S., Hernández-López J. (2008). Effect of Unilateral and Bilateral Eyestalk Ablation in Litopenaeus Vannamei Male and Female on Several Metabolic and Immunologic Variables. Aquaculture 283, 188–193. doi: 10.1016/j.aquaculture.2008.07.002
Sakaew W., Pratoomthai B., Anantasomboon G., Asuvapongpatana S., Sriurairattana S., Withyachumnarnkul B. (2008). Abdominal Segment Deformity Disease (ASDD) of the Whiteleg Shrimp Penaeus Vannamei Reared in Thailand. Aquaculture 284, 46–52. doi: 10.1016/j.aquaculture.2008.07.041
Sakaew W., Pratoomthai B., Pongtippatee P., Flegel T. W., Withyachumnarnkul B. (2013). Discovery and Partial Characterization of a non-LTR Retrotransposon That may be Associated With Abdominal Segment Deformity Disease (ASDD) in the Whiteleg Shrimp Penaeus (Litopenaeus) Vannamei. BMC Vet. Res. 9, 189. doi: 10.1186/1746-6148-9-189
Sandeman DCK, M., Harzsch S. (2016). “Adaptive Trends in Malacostracan Brain Form and Function Relate to Behavior,” in Nervous Systems and Control of Behavior (Natural History of Crustacea) Oxford. Ed. Derby C. (New York: Thiel TOUP), pp 11–pp 48.
Segner H., Sundh H., Buchmann K., Douxfils J., Sundell K. S., Mathieu C., et al. (2012). Health of Farmed Fish: Its Relation to Fish Welfare and its Utility as Welfare Indicator. Fish. Physiol. Biochem. 38, 85–105. doi: 10.1007/s10695-011-9517-9
Senapin S., Jaengsanong C., Phiwsaiya K., Prasertsri S., Laisutisan K., Chuchird N., et al. (2012). Infections of MRNV (Macrobrachium Rosenbergii Nodavirus) in Cultivated Whiteleg Shrimp Penaeus Vannamei in Asia. Aquaculture 338-341, 41–46. doi: 10.1016/j.aquaculture.2012.01.019
Senapin S., Phiwsaiya K., Gangnonngiw W., Briggs M., Sithigorngul P., Flegel T. W. (2013). Dual Infections of Imnv and MRNV in Cultivated Penaeus Vannamei From Indonesia. Aquaculture 372-375, 70–73. doi: 10.1016/j.aquaculture.2012.10.027
Sepici-Dinçel A., Alparslan Z. N., Benli AÇ. K., Selvi M., Sarıkaya R., Özkul İ. A., et al. (2013). Hemolymph Biochemical Parameters Reference Intervals and Total Hemocyte Counts of Narrow Clawed Crayfish Astacus Leptodactylus (Eschscholt). Ecol. Indic. 24, 305–309. doi: 10.1016/j.ecolind.2012.07.002
Shinn A. P. P., Griffith J., Trong D., Vu T. Q., Jiravanichpaisal N. T., P. Briggs M. (2018). Asian Shrimp Production and the Economic Costs of Disease. Asian Fish. Sci. 31S, 29–58. doi: 10.33997/j.afs.2018.31.S1.003
Smith J. A. (1991). A Question of Pain in Invertebrates. ILAR J. 33, 25–31. doi: 10.1093/ilar.33.1-2.25
Smith S. A., Berkson J. (2005). Laboratory Culture and Maintenance of the Horseshoe Crab (Limulus Polyphemus). Lab. Anim. 34, 27–34. doi: 10.1038/laban0705-27
Smith S. A., Berkson J. M., Barratt R. A. (2002). “Horseshoe Crab (Limulus Polyphemus) Hemolymph, Biochemical and Immunological Parameters,” in Proceedings of the 33rd Annual Conference of the International Association for Aquatic Animal Medicine Stamford, U.S.A.: International Association for Aquatic Animal Medicine Proceedings Online.
Smith J. A., Boyd K. M. (1991). “Lives in the Balance,” in The Ethics of Using Animals in Biomedical Research: The Report of a Working Party of the Institute of Medical Ethics. Eds. Smith J. A., Boyd K. M. (Oxford: Oxford university press).
Smith V. J., Chisholm J. R. S. (1992). Non-Cellular Immunity in Crustaceans. Fish. Shellfish Immunol. 2, 1–31. doi: 10.1016/S1050-4648(06)80024-0
Smith V. J., Ratcliffe N. A. (1980). Cellular Defense Reactions of the Shore Crab, Carcinus Maenas: In Vivo Hemocytic and Histopathological Responses to Injected Bacteria. J. Invertebr. Pathol. 35, 65–74. doi: 10.1016/0022-2011(80)90085-3
Smith V. J., Söderhäll K. (1983). Induction of Degranulation and Lysis of Haemocytes in the Freshwater Crayfish, Astacus Astacus by Components of the Prophenoloxidase Activating System In Vitro. Cell Tissue Res. 233, 295–303. doi: 10.1007/BF00238297
Söderhäll K., Smith V. J. (1983). Separation of the Haemocyte Populations of Carcinus Maenas and Other Marine Decapods, and Prophenoloxidase Distribution. Dev. Comp. Immunol. 7, 229–239. doi: 10.1016/0145-305X(83)90004-6
Sriurairatana S., Boonyawiwat V., Gangnonngiw W., Laosutthipong C., Hiranchan J., Flegel T. W. (2014). White Feces Syndrome of Shrimp Arises From Transformation, Sloughing and Aggregation of Hepatopancreatic Microvilli Into Vermiform Bodies Superficially Resembling Gregarines. PloS One 9, e99170. doi: 10.1371/journal.pone.0099170
Sterling P., Eyer J. (1988). “Allostasis: A New Paradigm to Explain Arousal Pathology,” in Handbook of Life Stress, Cognition and Health. Eds. Fisher S., Reason J. (Hoboken, U.S.A.: John Wiley & Sons), pp. 629–649.
Strausfeld N. J., Wolff G. H., Sayre M. E. (2020). Mushroom Body Evolution Demonstrates Homology and Divergence Across Pancrustacea. Elife 9, e52411. doi: 10.7554/eLife.52411.sa2
Suleiman S., Smith V. J., Dyrynda E. A. (2017). Unusual Tissue Distribution of Carcinin, an Antibacterial Crustin, in the Crab, Carcinus Maenas, Reveals its Multi-Functionality. Dev. Comp. Immunol. 76, 274–284. doi: 10.1016/j.dci.2017.06.010
Su X. P., Zhu B. S., Wang F. (2021). Feeding Strategy Changes Boldness and Agonistic Behaviour in the Swimming Crab (Portunus Trituberculatus). Aquac. Res. 53, 2, 419–430. doi: 10.1111/are.15583
Talbot P., Howard D., Leung-Trujillo J., Lee T. W., Li W. Y., Ro H., et al. (1989). Characterization of Male Reproductive Tract Degenerative Syndrome in Captive Penaeid Shrimp (Penaeus Setiferus). Aquaculture 78, 365–377. doi: 10.1016/0044-8486(89)90112-9
Tang K. F. J., Han J. E., Aranguren L. F., White-Noble B., Schmidt M. M., Piamsomboon P., et al. (2016). Dense Populations of the Microsporidian Enterocytozoon Hepatopenaei (EHP) in Feces of Penaeus Vannamei Exhibiting White Feces Syndrome and Pathways of Their Transmission to Healthy Shrimp. J. Invertebr. Pathol. 140, 1–7. doi: 10.1016/j.jip.2016.08.004
Tassanakajon A., Rimphanitchayakit V., Visetnan S., Amparyup P., Somboonwiwat K., Charoensapsri W., et al. (2018). Shrimp Humoral Responses Against Pathogens: Antimicrobial Peptides and Melanization. Dev. Comp. Immunol. 80, 81–93. doi: 10.1016/j.dci.2017.05.009
Taylor J., Vinatea L., Ozorio R., Schuweitzer R., Andreatta E. R. (2004). Minimizing the Effects of Stress During Eyestalk Ablation of Litopenaeus Vannamei Females With Topical Anesthetic and a Coagulating Agent. Aquaculture 233, 173–179. doi: 10.1016/j.aquaculture.2003.09.034
Thitamadee S., Prachumwat A., Srisala J., Jaroenlak P., Salachan P. V., Sritunyalucksana K., et al. (2016). Review of Current Disease Threats for Cultivated Penaeid Shrimp in Asia. Aquaculture 452, 69–87. doi: 10.1016/j.aquaculture.2015.10.028
Thomas M. M., Kathirvel M., Pillai N. N. (1974). Spawning and Rearing of the Penaeid Prawn, Metapenaeus Affinis (H. Milne Edwards) in the Laboratory. Indian J. Fish. 21, 543–556.
Tourtip S., Wongtripop S., Stentiford G. D., Bateman K. S., Sriurairatana S., Chavadej J., et al. (2009). Enterocytozoon Hepatopenaei Sp. Nov. (Microsporida: Enterocytozoonidae), a Parasite of the Black Tiger Shrimp Penaeus Monodon (Decapoda: Penaeidae): Fine Structure and Phylogenetic Relationships. J. Invertebr. Pathol. 102, 21–29. doi: 10.1016/j.jip.2009.06.004
Tran L., Nunan L., Redman R. M., Mohney L. L., Pantoja C. R., Fitzsimmons K., et al. (2013). Determination of the Infectious Nature of the Agent of Acute Hepatopancreatic Necrosis Syndrome Affecting Penaeid Shrimp. Dis. Aquat. Org. 105, 45–55. doi: 10.3354/dao02621
Treece G. D., Fox J. M. (1993). Design, Operation and Training Manual for an Intensive Culture Shrimp Hatchery With Emphasis on Penaeus Monodon and Penaeus Vannamei (Texas, U.S.A.: Texas ARM University Sea Grant College Program).
Treerattrakool S., Boonchoy C., Urtgam S., Panyim S., Udomkit A. (2014). Functional Characterization of Recombinant Gonad-Inhibiting Hormone (GIH) and Implication of Antibody Neutralization on Induction of Ovarian Maturation in Marine Shrimp. Aquaculture 428-429, 166–173. doi: 10.1016/j.aquaculture.2014.03.009
Tseng I. T., Chen J.-C. (2004). The Immune Response of White Shrimp Litopenaeus Vannamei and its Susceptibility to Vibrio Alginolyticus Under Nitrite Stress. Fish. Shellfish Immunol. 17, 325–333. doi: 10.1016/j.fsi.2004.04.010
Tumburu L., Shepard E. F., Strand A. E., Browdy C. L. (2012). Effects of Endosulfan Exposure and Taura Syndrome Virus Infection on the Survival and Molting of the Marine Penaeid Shrimp. Litopenaeus Vannamei Chemosphere 86, 912–918. doi: 10.1016/j.chemosphere.2011.10.057
Tu H. T., Silvestre F., Wang N., Thome J.-P., Phuong N. T., Kestemont P. (2010). A Multi-Biomarker Approach to Assess the Impact of Farming Systems on Black Tiger Shrimp (Penaeus Monodon). Chemosphere 81, 1204–1211. doi: 10.1016/j.chemosphere.2010.09.039
Uengwetwanit T., Pootakham W., Nookaew I., Sonthirod C., Angthong P., Sittikankaew K., et al. (2021). A Chromosome-Level Assembly of the Black Tiger Shrimp (Penaeus Monodon) Genome Facilitates the Identification of Growth-Associated Genes. Mol. Ecol. Resour. 21, 1620–1640. doi: 10.1111/1755-0998.13357
Utari H. B., Senapin S., Jaengsanong C., Flegel T. W., Kruatrachue M. (2012). A Haplosporidian Parasite Associated With High Mortality and Slow Growth in Penaeus (Litopenaeus Vannamei) Cultured in Indonesia. Aquaculture 366-367, 85–89. doi: 10.1016/j.aquaculture.2012.09.005
van de Braak C. B. T., Botterblom M. H. A., Taverne N., van Muiswinkel W. B., Rombout J. H. W. M., van der Knaap W. P. W. (2002). The Roles of Haemocytes and the Lymphoid Organ in the Clearance of Injected Vibrio Bacteria in Penaeus Monodon Shrimp. Fish. Shellfish Immunol. 13, 293–309. doi: 10.1006/fsim.2002.0409
van Hulten M. C. W., Witteveldt J., Snippe M., Vlak J. M. (2001). White Spot Syndrome Virus Envelope Protein Vp28 is Involved in the Systemic Infection of Shrimp. Virology 285, 228–233. doi: 10.1006/viro.2001.0928
Vargas-Albores F., Yepiz-Plascencia G. (2000). Beta Glucan Binding Protein and its Role in Shrimp Immune Response. Aquaculture 191, 13–21. doi: 10.1016/S0044-8486(00)00416-6
Verbruggen B. (2016). Generating Genomic Resources for Two Crustacean Species and Their Application to the Study of White Spot Disease (Exeter, UK: E-thesis University of Exeter). Available at: https://ethos.bl.uk/OrderDetails.do?uin=uk.bl.ethos.702288.
Villanueva R. R., Araneda M. E., Vela M., Seijo J. C. (2013). Selecting Stocking Density in Different Climatic Seasons: A Decision Theory Approach to Intensive Aquaculture. Aquaculture 384, 25–34. doi: 10.1016/j.aquaculture.2012.12.014
Wang Z. K., Luan S., Meng X. H., Cao B. X., Luo K., Kong J. (2019). Comparative Transcriptomic Characterization of the Eyestalk in Pacific White Shrimp (Litopenaeus Vannamei) During Ovarian Maturation. Gen. Comp. Endocrinol. 274, 60–72. doi: 10.1016/j.ygcen.2019.01.002
Wang G., Mcgaw I. J. (2014). Use of Serum Protein Concentration as an Indicator of Quality and Physiological Condition in the Lobster Homarus Americanus (Milne-Edward). J. Shellfish Res. 33 805-813, 809. doi: 10.2983/035.033.0315
Wang Q., Zhang B., Wang B. (2014). Effect Os Slurry Ice Treatment on the Quality of Fresh Litopenaeus Vannamei. Mod. Food Sci. Technol. 30, 134–140.
Wasielesky W., Froes C., Foes G., Krummenauer D., Lara G., Poersch L. (2013). Nursery of Litopenaeus Vannamei Reared in a Biofloc System: The Effect of Stocking Densities and Compensatory Growth. J. Shellfish Res. 32, 799–806. doi: 10.2983/035.032.0323
Weineck K., Ray A. J., Fleckenstein L. J., Medley M., Dzubuk N., Piana E., et al. (2018). Physiological Changes as a Measure of Crustacean Welfare Under Different Standardized Stunning Techniques: Cooling and Electroshock. Animals 8, 158. doi: 10.3390/ani8090158
Whetstone J. M. T., Treece G. D., Browdy C. L., Stokes A. D. (2002). Opportunities and Constraints in Marine Shrimp Farming. South. Reg. Aquac. Center 2006, 1–8.
Wijegoonawardane P. K. M., Cowley J. A., Phan T., Hodgson R. A. J., Nielsen L., Kiatpathomchai W., et al. (2008). Genetic Diversity in the Yellow Head Nidovirus Complex. Virology 380, 213–225. doi: 10.1016/j.virol.2008.07.005
Wu B., Zhao C. P., Xiong Z. T., Mu C. K., Xu S. L., Wang D. L. (2021). Analysis of the Agonistic Behaviour and Behavioural Pattern of Portunus Trituberculatus. Aquac. Res. 52 5, 2233–2242. doi: 10.1111/are.15075
Wycoff S. J., Weineck K., Conlin S., Suryadevara C., Grau E., Bradley A., et al. (2018). Effects of Clove Oil (Eugenol) on Proprioceptive Neurons, Heart Rate, and Behavior in Model Crustaceans. Biol. Fac. Publ. 145, 1–21.
Yano I., Kanna R. A., Oyama R. N., Wyban J. A. (1988). Mating Behaviour in the Penaeid Shrimp Penaeus Vannamei. Mar. Biol. 97, 171–175. doi: 10.1007/BF00391299
Yepiz-Plascencia G., Galván T. G., Vargas-albores F., García-bañuelos M. (2000). Synthesis of Hemolymph High-Density Lipoprotein [Beta]-Glucan Binding Protein by Penaeus Vannamei Shrimp Hepatopancreas. Mar. Biotechnol. 2, 485–492. doi: 10.1007/s101260000030
Zacarias S., Carboni S., Davie A., Little D. C. (2019). Reproductive Performance and Offspring Quality of non-Ablated Pacific White Shrimp (Litopenaeus Vannamei) Under Intensive Commercial Scale Conditions. Aquaculture 503, 460–466. doi: 10.1016/j.aquaculture.2019.01.018
Zacarias S., Fegan D., Wangsoontorn S., Yamuen N., Limakom T., Carboni S., et al. (2021). Increased Robustness of Postlarvae and Juveniles From non-Ablated Pacific Whiteleg Shrimp, Penaeus Vannamei, Broodstock Post-Challenged With Pathogenic Isolates of Vibrio Parahaemolyticus (VPAHPND) and White Spot Disease (WSD). Aquaculture 532, 736033. doi: 10.1016/j.aquaculture.2020.736033
Zhang Q., Liu Q., Liu S., Yang H., Liu S., Zhu L., et al. (2014). A New Nodavirus is Associated With Covert Mortality Disease of Shrimp. J. Gen. Virol. 95, 2700–2709. doi: 10.1099/vir.0.070078-0
Zhang Q., Xu T., Wan X., Liu S., Wang X., Li X., et al. (2017). Prevalence and Distribution of Covert Mortality Nodavirus (CMNV) in Cultured Crustacean. Virus Res. 233, 113–119. doi: 10.1016/j.virusres.2017.03.013
Zhang X., Yuan J., Sun Y., Li S., Gao Y., Yu Y., et al. (2019). Penaeid Shrimp Genome Provides Insights Into Benthic Adaptation and Frequent Molting. Nat. Commun. 10, 1–14. doi: 10.1038/s41467-018-08197-4
Keywords: sentience, penaeids, farming, welfare, disease, aquaculture, humane slaughter
Citation: Albalat A, Zacarias S, Coates CJ, Neil DM and Planellas SR (2022) Welfare in Farmed Decapod Crustaceans, With Particular Reference to Penaeus vannamei. Front. Mar. Sci. 9:886024. doi: 10.3389/fmars.2022.886024
Received: 28 February 2022; Accepted: 11 April 2022;
Published: 06 May 2022.
Edited by:
Yangfang Ye, Ningbo University, ChinaReviewed by:
Jitao Li, Chinese Academy of Fishery Sciences (CAFS), ChinaKhor Waiho, University of Malaysia Terengganu, Malaysia
Copyright © 2022 Albalat, Zacarias, Coates, Neil and Planellas. This is an open-access article distributed under the terms of the Creative Commons Attribution License (CC BY). The use, distribution or reproduction in other forums is permitted, provided the original author(s) and the copyright owner(s) are credited and that the original publication in this journal is cited, in accordance with accepted academic practice. No use, distribution or reproduction is permitted which does not comply with these terms.
*Correspondence: Amaya Albalat, amaya.albalat@stir.ac.uk